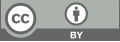
Emerging photocatalysts for efficient CO₂-to-Fuel conversion
- 1 Cogdel Cranleigh School Changsha, Hunan, China
* Author to whom correspondence should be addressed.
Abstract
Photocatalytic CO₂ reduction has emerged as a promising strategy to simultaneously mitigate climate change and meet growing energy needs by transforming CO₂ into useful fuels and chemicals using solar energy. This review systematically explores the key principles and latest progress in heterogeneous photocatalytic CO₂ conversion systems. The working mechanisms of CO₂ photoreduction are analyzed, with particular emphasis on high-performance photocatalysts responsive to both UV and visible light. To improve catalytic performance, various approaches for boosting the activity and product selectivity of these materials are discussed. Additionally, current challenges in photocatalytic CO₂ reduction are outlined, along with potential research directions to overcome these barriers. The findings presented here offer valuable insights for designing more effective photocatalytic systems, ultimately advancing CO₂ utilization technologies.
Keywords
photocatalyst, CO2 reduction, solar fuels, catalytic selectivity, catalyst design
[1]. Gust, D., Moore, T. A., & Moore, A. L. (2009). Solar Fuels via Artificial Photosynthesis. Accounts of Chemical Research, 42(12), 1890-1898.
[2]. Jia, J., Wang, H., Lu, Z., O'Brien, P. G., Ghoussoub, M., Duchesne, P., Zheng, Z., Li, P., Qiao, Q., & Wang, L. (2017). Photothermal Catalyst Engineering: Hydrogenation of Gaseous CO2 with High Activity and Tailored Selectivity. Advanced Science, 4(11), 1700252.
[3]. Lee, D., Yamauchi, K., & Sakai, K. (2024). Water-Induced Switching in Selectivity and Steric Control of Activity in Photochemical CO2 Reduction Catalyzed by RhCp*(bpy) Derivatives. Journal of the American Chemical Society, 146(82), 31597-31611.
[4]. Halmann, M. (1978). Photoelectrochemical Reduction of Aqueous Carbon Dioxide on p-type Gallium Phosphide in Liquid Junction Solar Cells. Nature, 275(5676), 115-116.
[5]. Wang, P., Meng, S., Zhang, B., He, M., Li, P., Yang, C., Li, G., & Li, Z. (2023). Sub-1 nm Cu2O Nanosheets for the Electrochemical CO2 Reduction and Valence State–Activity Relationship. Journal of the American Chemical Society, 145(49), 26133-26143.
[6]. Chen, J., Li, T., An, X., & Fu, D. (2024). Photocatalytic Reduction of CO2 by TiO2 Modified Materials: Recent Advances and Outlook. Energy & Fuels, 38(18), 7614-7636.
[7]. Abbas, T., Yahya, H. S. M., & Amin, N. A. S. (2023). Insights and Progress on Photocatalytic and Photoelectrocatalytic Reactor Configurations and Materials for CO2 Reduction to Solar Fuels. Energy & Fuels, 37(24), 18330-18368.
[8]. Goeppert, A., Czaun, M., Jones, J.-P., Surya Prakash, G. K., & Olah, G. A. (2014). Recycling of Carbon Dioxide to Methanol and Derived Products-Closing the Loop. Chemical Society Reviews, 43(22), 7995-8048.
[9]. Osterloh, F. E. (2017). Photocatalysis versus Photosynthesis: A Sensitivity Analysis of Devices for Solar Energy Conversion and Chemical Transformations. ACS Energy Letters, 2(3), 445-453.
[10]. Li, P., Hu, H., Luo, G., Zhu, S., Guo, L., Qu, P., Shen, Q., & He, T. (2020). Crystal Facet-Dependent CO2 Photoreduction over Porous ZnO Nanocatalysts. ACS Applied Materials & Interfaces, 12(50), 56039-56048.
[11]. Tang, Y., Zhu, X., Luo, Q., Fu, C.-F., & Li, X. (2025). Reversibly Modulating the Selectivity of Carbon Dioxide Reduction via Ligand-Driven Spin Crossover. The Journal of Physical Chemistry Letters, 16(1), 1-8.
[12]. Sun, J., Lian, G., Chen, Z., Zou, Z., & Wang, L. (2024). Merger of Single-Atom Catalysis and Photothermal Catalysis for Future Chemical Production. ACS Nano, 18(44), 34572-34595.
[13]. Wang, G., He, C. T., Huang, R., Mao, J., Wang, D., & Li, Y. (2020). Photoinduction of Cu Single Atoms Decorated on UiO-66-NH2 for Enhanced Photocatalytic Reduction of CO2 to Liquid Fuels. Journal of the American Chemical Society, 142(44), 19339-19345.
[14]. Cao, S., Shen, B., Tong, T., Fu, J., & Yu, J. (2018). 2D/2D Heterojunction of Ultrathin MXene/Bi2WO6 Nanosheets for Improved Photocatalytic CO2 Reduction. Advanced Functional Materials, 28(41), 1800136.
[15]. Zhang, H., Hong, Q., Li, J., Wang, F., Huang, X., Chen, S., Tu, W., Yu, D., Xu, R., Zhou, T., & Zhang, J. (2019). Isolated Square-Planar Copper Center in Boron Imidazolate Nanocages for Photocatalytic Reduction of CO2 to CO. Angewandte Chemie - International Edition, 58(34), 11752-11756.
[16]. Fang, J., Zhu, C., Hu, H., Li, J., Li, L., Zhu, H., & Mao, J. (2024). Progress of Photocatalytic CO2 Reduction toward Multi-carbon Products. Science China Chemistry, 67(22), 3994-4013.
[17]. Osterloh, F. E. (2017). Photocatalysis Versus Photosynthesis: A Sensitivity Analysis of Devices for Solar Energy Conversion and Chemical Transformations. ACS Energy Letters, 2(3), 445-453.
[18]. Adekoya, D., Tahir, M., & Amin, N. A. S. (2019). Recent trends in photocatalytic materials for reduction of carbon dioxide to methanol. Renewable and Sustainable Energy Reviews, 116, 109389.
[19]. Lin, J., Tian, W., Zhang, H., Duan, X., Sun, H., & Wang, S. (2021). Graphitic carbon nitride-based Z-scheme structure for photocatalytic CO2 reduction. Energy & Fuels, 35(1), 7-24.
[20]. Ran, J., Jaroniec, M., & Qiao, S. (2018). Cocatalysts in Semiconductor-Based Photocatalytic CO2 Reduction: Achievements, Challenges, and Opportunities. Advanced Materials, 30(41), 1704649.
[21]. Lei, B., Cui, W., Chen, P., Chen, L., Li, J., & Dong, F. (2022). C–Doping Induced Oxygen-Vacancy in WO3 Nanosheets for CO2 Activation and Photoreduction. ACS Catalysis, 12(14), 9670-9678.
[22]. Adachi, Y., Turanský, R., Brndiar, J., Tokár, K., Zhu, Q., Wen, H. F., Sugawara, Y., Štich, I., & Li, Y. J. (2024). Mechanocatalysis of CO to CO2 on TiO2 Surface Controlled at Atomic Scale. Nano Research, 17(19), 5826-5834.
[23]. Shen, Q., Chen, Z., Huang, X., Liu, M., & Zhao, G. (2015). High-Yield and Selective Photoelectrocatalytic Reduction of CO2 to Formate by Metallic Copper Decorated Co3O4 Nanotube Arrays. Environmental Science & Technology, 49(10), 5828-5835.
[24]. Lin, X., Wang, Z. T., Lyubinetsky, I., Kay, B. D., & Dohnalek, Z. (2013). Interaction of CO2 with Oxygen Adatoms on Rutile TiO2(110). Physical Chemistry Chemical Physics, 15(23), 6190-6195.
[25]. Zhu, S., Guo, L., Li, P., Zhang, B., Zhao, G., & He, T. (2019). A Computational Study on Linear and Bent Adsorption of CO2 on Different Surfaces for Its Photoreduction. Catalysis Today, 335, 278-285.
[26]. Mino, L., Spoto, G., & Ferrari, A. M. (2014). CO2 Capture by TiO2 Anatase Surfaces: A Combined DFT and FTIR Study. Journal of Physical Chemistry C, 118(45), 25016-25026.
[27]. Yoo, J. S., Christensen, R., Vegge, T., Norskov, J. K., & Studt, F. (2016). Theoretical Insight into the Trends That Guide the Electrochemical Reduction of Carbon Dioxide to Formic Acid. ChemSusChem, 9(3), 358-363.
[28]. Luo, W. J., Nie, X. W., Janik, M. J., & Asthagiri, A. (2016). Facet Dependence of CO2 Reduction Paths on Cu Electrodes. ACS Catalysis, 6(4), 219-229.
[29]. Man, S., Jiang, W., Guo, X., Ruzimuradov, O., Mamatkulov, S., Low, J., & Xiong, Y. (2024). Materials Design for Photocatalytic CO2 Conversion to C2+ Products. Chemical Materials, 36(6), 1793-1809.
[30]. Tang, J., Durrant, J. R., & Klug, D. R. (2008). Mechanism of Photocatalytic Water Splitting in TiO2. Reaction of Water with Photoholes, Importance of Charge Carrier Dynamics, and Evidence for Four-Hole Chemistry. Journal of the American Chemical Society, 130(42), 13885-13891.
[31]. Chang, X., Wang, T., & Gong, J. (2016). CO2 Photo-Reduction: Insights into CO2 Activation and Reaction on Surfaces of Photocatalysts. Energy & Environmental Science, 9(6), 2177-2196.
[32]. Albero, J., Peng, Y., & García, H. (2020). Photocatalytic CO2 Reduction to C2+ Products. ACS Catalysis, 10(12), 5734-5749.
[33]. Wang, T., Chen, L., Chen, C., Huang, M., Huang, Y., Liu, S., & Li, B. (2022). Engineering Catalytic Interfaces in Cuδ+/CeO2-TiO2 Photocatalysts for Synergistically Boosting CO2 Reduction to Ethylene. ACS Nano, 16(3), 2306-2318.
[34]. Zhu, H., Guo, R., Liu, C., Cui, H., Liu, M., & Pan, W. (2024). Recent Progress on Photocatalytic Reduction of CO2 to C2+ products. Journal of Materials Chemistry A, 12(47), 21677-21703.
[35]. Yu, J. G., Low, J. X., Xiao, W., Zhou, P., & Jaroniec, M. (2014). Enhanced Photocatalytic CO2-Reduction Activity of Anatase TiO2 by Coexposed {001} and {101} Facets. Journal of the American Chemical Society, 136(25), 8839-8842.
[36]. Low, J., Zhang, L., Zhu, B., Liu, Z., & Yu, J. (2018). TiO2 Photonic Crystals with Localized Surface Photothermal Effect and Enhanced Photocatalytic CO2 Reduction Activity. ACS Sustainable Chemistry & Engineering, 6(12), 15653-15661.
[37]. Kuehnel, M. F., Orchard, K. L., Dalle, K. E., & Reisner, E. (2017). Selective Photocatalytic CO2 Reduction in Water through Anchoring of a Molecular Ni Catalyst on CdS Nanocrystals. Journal of the American Chemical Society, 139(21), 7217-7223.
[38]. Li, X. D., Sun, Y. F., Xu, J. Q., Shao, Y. J., Wu, J., Xu, X. L., Pan, Y., Ju, H. X., Zhu, J. F., & Xie, Y. (2019). Selective Visible-light-driven Photocatalytic CO2 Reduction to CH4 Mediated by Atomically Thin CuIn5S8 Layers. Nature Energy, 4(8), 690-699.
[39]. Ye, W., Guo, X., & Ma, T. (2021). A Review on Electrochemical Synthesized Copper-Based Catalysts for Electrochemical Reduction of CO2 to C2+ Products. Chemical Engineering Journal, 414, 128825.
[40]. Bai, S. J., Jing, W. H., He, G. W., Liao, C., Wang, F., Liu, Y., & Guo, L. J. (2023). Near-Infrared-Responsive Photocatalytic CO2 Conversion via Generated Co3O4/Cu2O. ACS Nano, 17(12), 10976-10986.
[41]. Chen, Z., Hu, Y., Wang, J., Shen, Q., Zhang, Y., Ding, C., Bai, Y., Jiang, G., Li, Z., & Gaponik, N. (2020). Boosting Photocatalytic CO2 Reduction on CsPbBr3 Perovskite Nanocrystals by Immobilizing Metal Complexes. Chemical Materials, 32(4), 1517-1525.
[42]. Hai, Y., Huang, W., Li, Z., Wu, D., Huang, Q., & Tang, X. (2021). Morphology Regulation and Photocatalytic CO2 Reduction of Lead-Free Perovskite Cs3Sb2I9 Microcrystals. ACS Applied Energy Materials, 4(6), 5913-5917.
[43]. Li, N. Y., Chen, X. J., Wang, J., Liang, X. M., Ma, L. T., Jing, X. L., Chen, D. L., & Li, Z. Q. (2022). ZnSe Nanorods-CsSnCl3 Perovskite Heterojunction Composite for Photocatalytic CO2 Reduction. ACS Nano, 16(4), 3332-3340.
[44]. Ban, C. G., Wang, Y., Feng, Y. J., Zhu, Z. H., Duan, Y. Y., Ma, J. P., Zhang, X., Liu, X., Zhou, K., Zou, H. J., Yu, D. M., Tao, X. P., Gan, L. Y., Han, G., & Zhou, X. Y. (2024). Photochromic Single Atom Ag/TiO2 Catalysts for Selective CO2 Reduction to CH4 Proton Turnover Dominated Cascade Route for CO2 Photoreduction. Energy & Environmental Science, 17(2), 518-530.
[45]. Fang, J., Li, J., Chen, Y., Cheng, J., Zhu, C., & Mao, J. (2024). Au Cluster–nanoparticle Dual Coupling for Photocatalytic CO2 Conversion. Inorganic Chemistry, 63(45), 19375-19381.
[46]. Chai, Y., Kong, Y. H., Lin, M., Lin, W., Shen, J. N., Long, J. L., Yuan, R. S., Dai, W. X., Wang, X. X., & Zhang, Z. Z. (2023). Metal to Non-metal Sites of Metallic Sulfides Switching Products from CO to CH4 for Photocatalytic CO2 Reduction. Nature Communications, 14(1), 6168.
[47]. Soontornchaiyakul, W., Yoshino, S., Kanazawa, T., Haruki, R., Fan, D. X., Nozawa, S., Yamaguchi, Y., & Kudo, A. (2023). CH4 Synthesis from CO2 and H2O of an Electron Source over Rh-Ru Cocatalysts Loaded on NaTaO3:Sr Photocatalysts. Journal of the American Chemical Society, 145(44), 20485-20491.
[48]. Varghese, O. K., Paulose, M., LaTempa, T. J., & Grimes, C. A. (2009). High-Rate Solar Photocatalytic Conversion of CO2 and Water Vapor to Hydrocarbon Fuels. Nano Letters, 9(2), 731-737.
[49]. Li, X., Yu, J., Jaroniec, M., & Chen, X. (2019). Cocatalysts for Selective Photoreduction of CO2 into Solar Fuels. Chemical Reviews, 119(6), 3962-4
[50]. Li, K., Peng, B., & Peng, T. (2016). Recent Advances in Heterogeneous Photocatalytic CO2 Conversion to Solar Fuels.ACS Catalysis, 6(11), 7485-7527.
[51]. Kong, M., Li, Y., Chen, X., Tian, T., Fang, P., Zheng, F., & Zhao, X. (2011). Tuning the Relative Concentration Ratio of Bulk Defects to Surface Defects in TiO2 Nanocrystals Leads to High Photocatalytic Efficiency.Journal of the American Chemical Society, 133(42), 16414-16417.
[52]. Luo, L., Fu, L., Liu, H. F., Xu, Y. X., Xing, J. L., Chang, C. R., Yang, D. Y., & Tang, J. W. (2022). Synergy of Pd Atoms and Oxygen Vacancies on In2O3 for Methane Conversion under Visible Light.Nature Communications, 13(1), 2930.
[53]. Yang, S. D., Guo, X., Li, X. M., Wu, T. Z., Zou, L. H., He, Z. Y., Xu, Q., Zheng, J. J., Chen, L., Wang, Q. Y., & Xu, Z. C. J. (2024). Enhancing Photocatalytic CO2 Conversion through Oxygen-vacancy-mediated Topological Phase Transition. Angewandte Chemie - International Edition, 63(11), e202317957.
[54]. Dai, W., Long, J., Yang, L., Zhang, S., Xu, Y., Luo, X., Zou, J., & Luo, S. (2021). Oxygen Migration Triggering Molybdenum Exposure in Oxygen Vacancy-Rich Ultra-Thin Bi2MoO6 Nanoflakes: Dual Binding Sites Governing Selective CO2 Reduction into Liquid Hydrocarbons. Journal of Energy Chemistry, 61, 281-289.
Cite this article
Yi,J. (2025). Emerging photocatalysts for efficient CO₂-to-Fuel conversion. Advances in Engineering Innovation,16(4),51-59.
Data availability
The datasets used and/or analyzed during the current study will be available from the authors upon reasonable request.
Disclaimer/Publisher's Note
The statements, opinions and data contained in all publications are solely those of the individual author(s) and contributor(s) and not of EWA Publishing and/or the editor(s). EWA Publishing and/or the editor(s) disclaim responsibility for any injury to people or property resulting from any ideas, methods, instructions or products referred to in the content.
About volume
Volume title:
© 2024 by the author(s). Licensee EWA Publishing, Oxford, UK. This article is an open access article distributed under the terms and
conditions of the Creative Commons Attribution (CC BY) license. Authors who
publish this series agree to the following terms:
1. Authors retain copyright and grant the series right of first publication with the work simultaneously licensed under a Creative Commons
Attribution License that allows others to share the work with an acknowledgment of the work's authorship and initial publication in this
series.
2. Authors are able to enter into separate, additional contractual arrangements for the non-exclusive distribution of the series's published
version of the work (e.g., post it to an institutional repository or publish it in a book), with an acknowledgment of its initial
publication in this series.
3. Authors are permitted and encouraged to post their work online (e.g., in institutional repositories or on their website) prior to and
during the submission process, as it can lead to productive exchanges, as well as earlier and greater citation of published work (See
Open access policy for details).