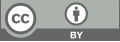
Energy recovery in automotive systems: A review of regenerative braking, flywheel, thermoelectric, rankin cycle and electric turbo compound
- 1 Nanjing tech University
- 2 Guangzhou city university of Technology
- 3 Zhengzhou Foreign Language School
- 4 Shanghai Star River Bay Bilingual School
- 5 Veritas Collegiate Academy
* Author to whom correspondence should be addressed.
Abstract
In the backdrop of escalating global vehicle proliferation and the consequent surge in carbon dioxide (CO2) emissions, there is a compelling urgency to innovate energy recovery methods during vehicular operations for heightened energy efficiency and substantial environmental emission reductions. This paper discussed a variety of vehicle technologies to address environmental emissions issues and critically evaluates contemporary energy recovery technologies across three core dimensions: automotive thermal energy conversion, electronic turbine systems (ETC), and kinetic energy recovery systems. By analyzing empirical data encompassing CO2 emission reduction, energy recovery efficiency enhancement, fuel economy improvement, and applicability diversity across vehicle models, the study aims to establish a scientific foundation for continuous vehicular energy efficiency enhancement and emission reduction. This entails a comprehensive investigation of internal combustion engine principles for potential heat energy recovery, juxtaposing conventional and advanced cycles like the Rankine cycle. Moreover, it involves assessing ETC effectiveness in utilizing exhaust energy while acknowledging system complexity and dynamic response challenges. Additionally, the performance disparities of kinetic energy recovery systems like regenerative braking and exhaust energy recovery in distinct operational contexts are examined. By meticulously comparing experimental data, the paper intends to provide a comprehensive assessment of each technology’s merits and drawbacks in advancing energy efficiency and emission reduction, try to pick one or more combinations to minimise the impact, thereby guiding future research and application in automotive energy recovery, and contributing to sustainable automotive engineering technology advancement.
Keywords
Rankine cycle, ETC, Thermoelectric, Energy recovery system
[1]. T. Han, B. Zeng, and Y. Tong, “Theoretical study on energy recovery rate of regenerative braking for hybrid mining trucks with different parameters,” J. Energy Storage, vol. 42, p. 103127, Oct. 2021, doi: 10.1016/j.est.2021.103127.
[2]. W.-H. Chen, T.-H. Huang, G. L. Augusto, R. Lamba, C. Maduabuchi, and L. H. Saw, “Power generation and thermal stress characterization of thermoelectric modules with different unileg couples by recovering vehicle waste heat,” J. Clean. Prod., vol. 375, p. 133987, Nov. 2022, doi: 10.1016/j.jclepro.2022.133987.
[3]. L. Zhang and X. Cai, “Control strategy of regenerative braking system in electric vehicles,” Energy Procedia, vol. 152, pp. 496–501, Oct. 2018, doi: 10.1016/j.egypro.2018.09.200.
[4]. S. Bai and C. Liu, “Overview of energy harvesting and emission reduction technologies in hybrid electric vehicles,” Renew. Sustain. Energy Rev., vol. 147, p. 111188, Sep. 2021, doi: 10.1016/j.rser.2021.111188.
[5]. Q. He, Y. Yang, C. Luo, J. Zhai, R. Luo, and C. Fu, “Energy recovery strategy optimization of dual-motor drive electric vehicle based on braking safety and efficient recovery,” Energy, vol. 248, p. 123543, Jun. 2022, doi: 10.1016/j.energy.2022.123543.
[6]. F. Atkins, “18 - Integration and performance of regenerative braking and energy recovery technologies in vehicles*,” in Alternative Fuels and Advanced Vehicle Technologies for Improved Environmental Performance (Second Edition), R. Folkson and S. Sapsford, Eds., in Woodhead Publishing Series in Energy. Woodhead Publishing, 2022, pp. 545–568. doi: 10.1016/B978-0-323-90979-2.00005-6.
[7]. D. Yadav, R. Kumar, U. Kulshrestha, A. Jain, and S. Rani, “Enhancement of fuel efficiency in heavy duty vehicles through integrated module of TEG, piezoelectric and regenerative braking solutions,” Mater. Today Proc., vol. 63, pp. 1–5, Jan. 2022, doi: 10.1016/j.matpr.2021.10.372.
[8]. F. Khajvand, M. Zareinejad, S. Mehdi Rezaei, and K. Baghestan, “Design and implementation of a series hydraulic hybrid propulsion system to increase regenerative braking energy saving range,” Energy Convers. Manag., vol. 279, p. 116754, Mar. 2023, doi: 10.1016/j.enconman.2023.116754.
[9]. Boretti, “Kinetic Energy Recover Systems for Racing Cars,” in Kinetic Energy Recovery Systems for Racing Cars, SAE, 2013, pp. i–v. Accessed: Aug. 06, 2023. [Online]. Available: https://ieeexplore.ieee.org/document/8505601
[10]. R. Kapoor and C. M. Parveen, “Comparative Study on Various KERS,” 2013.
[11]. T. Mathews, “FLYWHEEL BASED KINETIC ENERGY RECOVERY SYSTEMS (KERS) INTEGRATED IN VEHICLES,” Int. J. Eng. Sci. Technol., vol. 5, 2013.
[12]. Buchroithner, I. Andrasec, and M. Bader, “Optimal system design and ideal application of flywheel energy storage systems for vehicles,” in 2012 IEEE International Energy Conference and Exhibition (ENERGYCON), Florence, Italy: IEEE, Sep. 2012, pp. 991–996. doi: 10.1109/EnergyCon.2012.6348295.
[13]. M. Gee and R. W. Dunn, “Analysis of Trackside Flywheel Energy Storage in Light Rail Systems,” IEEE Trans. Veh. Technol., vol. 64, no. 9, pp. 3858–3869, Sep. 2015, doi: 10.1109/TVT.2014.2361865.
[14]. “Volvo Cars’ tests of flywheel technology confirm fuel savings of up to 25%,” Green Car Congress. https://www.greencarcongress.com/2013/04/kers-20130425.html (accessed Aug. 06, 2023).
[15]. Bamisile, Z. Zheng, H. Adun, D. Cai, N. Ting, and Q. Huang, “Development and prospect of flywheel energy storage technology: A citespace-based visual analysis,” Energy Rep., vol. 9, pp. 494–505, Oct. 2023, doi: 10.1016/j.egyr.2023.05.147.
[16]. S. J. Kim, K. Hayat, S. U. Nasir, and S. K. Ha, “Design and fabrication of hybrid composite hubs for a multi-rim flywheel energy storage system,” Compos. Struct., vol. 107, pp. 19–29, Jan. 2014, doi: 10.1016/j.compstruct.2013.07.032.
[17]. V. Kale and M. Secanell, “A comparative study between optimal metal and composite rotors for flywheel energy storage systems,” Energy Rep., vol. 4, pp. 576–585, Nov. 2018, doi: 10.1016/j.egyr.2018.09.003.
[18]. Murakami, Y. Zhao, and T. Tashiro, “Stabilization of a Magnetic Repulsive Levitation Flywheel System Using a High-Efficiency Superconducting Magnetic Bearing,” Actuators, vol. 11, no. 7, Art. no. 7, Jul. 2022, doi: 10.3390/act11070180.
[19]. D. Zi, L. Zhang, B. Chen, and Q. Zhang, “Study of the electric-booster and turbo-generator system and its influence on a 1.5 L gasoline engine,” Appl. Therm. Eng., vol. 162, p. 114236, Nov. 2019, doi: 10.1016/j.applthermaleng.2019.114236.
[20]. M. Yang et al., “Matching method of electric turbo compound for two-stroke low-speed marine diesel engine,” Appl. Therm. Eng., vol. 158, p. 113752, Jul. 2019, doi: 10.1016/j.applthermaleng.2019.113752.
[21]. P. Novotný, P. Kudláček, and J. Vacula, “Explanation of the mechanisms of unsteady gas flow through the turbocharger seal system, including thermal and structural interactions,” Propuls. Power Res., vol. 12, no. 2, pp. 180–198, Jun. 2023, doi: 10.1016/j.jppr.2023.05.003.
[22]. Arsie, A. Cricchio, C. Pianese, V. Ricciardi, and M. D. Cesare, “Evaluation of CO2 reduction in SI engines with Electric Turbo-Compound by dynamic powertrain modelling,” IFAC-Pap., vol. 48, no. 15, pp. 93–100, Jan. 2015, doi: 10.1016/j.ifacol.2015.10.014.
[23]. Arsie, A. Cricchio, C. Pianese, V. Ricciardi, and M. De Cesare, “Modeling Analysis of Waste Heat Recovery via Thermo-Electric Generator and Electric Turbo-Compound for CO2 Reduction in Automotive SI Engines,” Energy Procedia, vol. 82, pp. 81–88, Dec. 2015, doi: 10.1016/j.egypro.2015.11.886.
[24]. García, J. Monsalve-Serrano, S. Martinez-Boggio, and P. Gaillard, “Emissions reduction by using e-components in 48 V mild hybrid trucks under dual-mode dual-fuel combustion,” Appl. Energy, vol. 299, p. 117305, Oct. 2021, doi: 10.1016/j.apenergy.2021.117305.
[25]. Mohd Noor, R. Che Puteh, S. Rajoo, U. M. Basheer, M. H. Md Sah, and S. H. Shaikh Salleh, “Simulation Study on Electric Turbo-Compound (ETC) for Thermal Energy Recovery in Turbocharged Internal Combustion Engine,” Appl. Mech. Mater., vol. 799–800, pp. 895–901, Oct. 2015, doi: 10.4028/www.scientific.net/AMM.799-800.895.
[26]. W. Wei, W. Zhuge, Y. Zhang, and Y. He, “Comparative Study on Electric Turbo-Compounding Systems for Gasoline Engine Exhaust Energy Recovery,” in Volume 5: Industrial and Cogeneration; Microturbines and Small Turbomachinery; Oil and Gas Applications; Wind Turbine Technology, Glasgow, UK: ASMEDC, Oct. 2010, pp. 531–539. doi: 10.1115/GT2010-23204.
[27]. M. Algrain, “Controlling an Electric Turbo Compound System for Exhaust Gas Energy Recovery in a Diesel Engine Marcelo C. Algrain,”.
[28]. E. Pipitone and S. Caltabellotta, “The Potential of a Separated Electric Compound Spark-Ignition Engine for Hybrid Vehicle Application,” J. Eng. Gas Turbines Power, vol. 144, no. 4, p. 041016, Apr. 2022, doi: 10.1115/1.4053393.
[29]. W. Zhuge, L. Huang, W. Wei, Y. Zhang, and Y. He, “Optimization of an Electric Turbo Compounding System for Gasoline Engine Exhaust Energy Recovery,” presented at the SAE 2011 World Congress & Exhibition, Apr. 2011, pp. 2011-01–0377. doi: 10.4271/2011-01-0377.
[30]. M. Umar, H. Nozari, M. R. Menezes, J. M. Herreros, C. S. Lau, and A. Tsolakis, “Hydrogen production from bioethanol fuel mixtures via exhaust heat recovery in diesel engines: A promising route towards more energy efficient road vehicles,” Int. J. Hydrog. Energy, vol. 46, no. 46, Art. no. 46, Jul. 2021, doi: 10.1016/j.ijhydene.2021.04.146.
[31]. N. Kempf and Y. Zhang, “Design and optimization of automotive thermoelectric generators for maximum fuel efficiency improvement,” Energy Convers. Manag., vol. 121, pp. 224–231, Aug. 2016, doi: 10.1016/j.enconman.2016.05.035.
[32]. T. Morimura, M. Hasaka, and M. Yoshimoto, “TEM observation of coexistent Heusler and half-Heusler phases in TiNi1.5Sn,” J. Alloys Compd., vol. 416, no. 1, Art. no. 1, Jun. 2006, doi: 10.1016/j.jallcom.2005.08.043.
[33]. T. Yamamoto, T. Furuhata, N. Arai, and K. Mori, “Design and testing of the Organic Rankine Cycle,” Energy, vol. 26, no. 3, pp. 239–251, Mar. 2001, doi: 10.1016/S0360-5442(00)00063-3.
[34]. Boretti, “Recovery of exhaust and coolant heat with R245fa organic Rankine cycles in a hybrid passenger car with a naturally aspirated gasoline engine,” Appl. Therm. Eng., vol. 36, pp. 73–77, Apr. 2012, doi: 10.1016/j.applthermaleng.2011.11.060.x
Cite this article
Shu,C.;Cao,Y.;Sun,T.;Zhang,T.;Wang,X. (2024). Energy recovery in automotive systems: A review of regenerative braking, flywheel, thermoelectric, rankin cycle and electric turbo compound. Applied and Computational Engineering,85,44-60.
Data availability
The datasets used and/or analyzed during the current study will be available from the authors upon reasonable request.
Disclaimer/Publisher's Note
The statements, opinions and data contained in all publications are solely those of the individual author(s) and contributor(s) and not of EWA Publishing and/or the editor(s). EWA Publishing and/or the editor(s) disclaim responsibility for any injury to people or property resulting from any ideas, methods, instructions or products referred to in the content.
About volume
Volume title: Proceedings of the 4th International Conference on Materials Chemistry and Environmental Engineering
© 2024 by the author(s). Licensee EWA Publishing, Oxford, UK. This article is an open access article distributed under the terms and
conditions of the Creative Commons Attribution (CC BY) license. Authors who
publish this series agree to the following terms:
1. Authors retain copyright and grant the series right of first publication with the work simultaneously licensed under a Creative Commons
Attribution License that allows others to share the work with an acknowledgment of the work's authorship and initial publication in this
series.
2. Authors are able to enter into separate, additional contractual arrangements for the non-exclusive distribution of the series's published
version of the work (e.g., post it to an institutional repository or publish it in a book), with an acknowledgment of its initial
publication in this series.
3. Authors are permitted and encouraged to post their work online (e.g., in institutional repositories or on their website) prior to and
during the submission process, as it can lead to productive exchanges, as well as earlier and greater citation of published work (See
Open access policy for details).