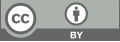
Advancements in shape-memory alloys: Properties, applications, challenges, and future prospects
- 1 Shanghai Pinghe School, Shanghai, China
* Author to whom correspondence should be addressed.
Abstract
Shape-memory alloys (SMAs) are advanced engineering materials that have gained significant attention in recent years due to their unique properties and potential applications. SMAs have the remarkable ability to recover their original shape after deformation, making them invaluable in various fields, from biomedical devices to aerospace engineering. Despite their many advantages, SMAs also face several challenges, including the need for improved processing techniques and the development of more efficient actuation systems. To address these challenges, researchers have adopted various approaches, including using advanced fabrication methods and developing novel actuation systems. Recent research has yielded several notable achievements in the field of SMAs. For example, researchers have developed new processing techniques that allow the production of SMAs with improved properties, such as higher strength and better fatigue resistance. Additionally, researchers have developed new actuation systems that allow for more precise and efficient control of SMA behavior. Looking ahead, the future of SMAs looks promising. With continued research and development, SMAs have the potential to revolutionize various fields, from aerospace engineering to biomedical devices. However, further work is needed to overcome the remaining challenges and fully realize the potential of these remarkable materials. This article provides a comprehensive overview of SMAs, including their properties, fabrication methods, and various applications. It also discusses the challenges facing the field, the approaches to address them, and recent achievements.
Keywords
Shape-memory alloys, advanced engineering materials
[1]. U. Dahmen, “Phase Transformations, Crystallographic Aspects,” in Encyclopedia of Physical Science and Technology, 2003. doi: 10.1016/b0-12-227410-5/00560-3.
[2]. K. K. Alaneme and E. A. Okotete, “Reconciling viability and cost-effective shape memory alloy options – A review of copper and iron based shape memory metallic systems,” 2016. doi: 10.1016/j.jestch.2016.05.010.
[3]. D. J. Fernandes, R. V. Peres, A. M. Mendes, and C. N. Elias, “Understanding the Shape-Memory Alloys Used in Orthodontics,” ISRN Dent, vol. 2011, 2011, doi: 10.5402/2011/132408.
[4]. C. Song, “History and Current Situation of Shape Memory Alloys Devices for Minimally Invasive Surgery,” The Open Medical Devices Journal, vol. 2, no. 2, 2010, doi: 10.2174/1875181401002020024.
[5]. D. J. Hoh, B. L. Hoh, A. P. Amar, and M. Y. Wang, “Shape memory alloys: Metallurgy, biocompatibility, and biomechanics for neurosurgical applications,” Neurosurgery, vol. 64, no. SUPPL. 5, 2009, doi: 10.1227/01.NEU.0000330392.09889.99.
[6]. L. Petrini and F. Migliavacca, “Biomedical Applications of Shape Memory Alloys,” Journal of Metallurgy, vol. 2011, 2011, doi: 10.1155/2011/501483.
[7]. R. Pecora, I. Dimino, M. Bray, and R. Bray, “SMA for aeronautics,” in Shape Memory Alloy Engineering: For Aerospace, Structural, and Biomedical Applications, 2021. doi: 10.1016/B978-0-12-819264-1.00015-7.
[8]. L. Janke, C. Czaderski, M. Motavalli, and J. Ruth, “Applications of shape memory alloys in civil engineering structures - Overview, limits and new ideas,” Materials and Structures/Materiaux et Constructions, vol. 38, no. 279, 2005, doi: 10.1617/14323.
[9]. A. Concilio, V. Antonucci, F. Auricchio, L. Lecce, and E. Sacco, Shape Memory Alloy Engineering: For Aerospace, Structural, and Biomedical Applications. 2021. doi: 10.1016/B978-0-12-819264-1.01001-3.
[10]. G. Song and N. Ma, “Shape memory alloy actuated adaptive exhaust nozzle for jet engine,” 2009
[11]. A. Guzik and O. Benafan, “Design and Development of CubeSat Solar Array Deployment Mechanisms Using Shape Memory Alloys,” in 44th Aerospace Mechanisms Symposium, 2018.
[12]. J. M. Llanto, M. Tolouei-Rad, A. Vafadar, and M. Aamir, “Recent progress trend on abrasive waterjet cutting of metallic materials: A review,” 2021. doi: 10.3390/app11083344.
[13]. M. Muniraju and G. Talla, “Exploring sustainable machining processes for nitinol shape memory alloy: a review of eco-friendly EDM and other techniques,” 2024. doi: 10.1007/s40430-023-04642-8.
[14]. M. C. Kong, D. Axinte, and W. Voice, “Challenges in using waterjet machining of NiTi shape memory alloys: An analysis of controlled-depth milling,” J Mater Process Technol, vol. 211, no. 6, 2011, doi: 10.1016/j.jmatprotec.2010.12.015.
[15]. X. Liu, Z. Liang, G. Wen, and X. Yuan, “Waterjet machining and research developments: a review,” 2019. doi: 10.1007/s00170-018-3094-3.
[16]. P. Thakur, D. N. Raut, and F. Siddiqui, “Recent Applications, Developments and Challenges in Waterjet Technology,” in Lecture Notes in Mechanical Engineering, 2022. doi: 10.1007/978-981-16-9236-9_14.
[17]. H. T. P. Liu, “Advanced waterjet technology for machining curved and layered structures,” Curved and Layered Structures, vol. 6, no. 1, 2019, doi: 10.1515/cls-2019-0004.
[18]. J. W. Sohn, J. S. Ruth, D. G. Yuk, and S. B. Choi, “Application of Shape Memory Alloy Actuators to Vibration and Motion Control of Structural Systems: A Review,” 2023. doi: 10.3390/app13020995.
[19]. A. Dana, S. Vollach, and D. Shilo, “Use the force: review of high-rate actuation of shape memory alloys,” 2021. doi: 10.3390/act10070140.
[20]. S. Jayachandran, N. Yashwanth, H. F. Hussain, and I. A. Palani, “Investigation on Actuation Performance of Shape Memory Alloy Actuator for Robotics Application,” in Lecture Notes in Mechanical Engineering, 2023. doi: 10.1007/978-981-19-3866-5_45.
[21]. F. Taylor and C. K. Au, “Forced Air Cooling of Shape-Memory Alloy Actuators for a Prosthetic Hand,” J Comput Inf Sci Eng, vol. 16, no. 4, 2016, doi: 10.1115/1.4033233.
[22]. A. Osorio Salazar, Y. Sugahara, D. Matsuura, and Y. Takeda, “A Novel, Scalable Shape Memory Alloy Actuator Controlled by Fluid Temperature,” in Mechanisms and Machine Science, 2021. doi: 10.1007/978-3-030-55807-9_69.
[23]. S. Lederlé, “Issues in the design of shape memory alloy actuators,” Aeronatics and astronautics, 2002.
[24]. I. Y. Paez-Pidiache, A. Luviano-Juárez, N. Lozada-Castillo, E. Castillo-Castañeda, and H. Reyes-Torres, “Design, characterization and construction of an actuator based on shape memory alloys,” MRS Adv, vol. 6, no. 39–40, 2021, doi: 10.1557/s43580-021-00158-2.
[25]. R. W. Wheeler et al., “Engineering design tools for shape memory alloy actuators: CASMART collaborative best practices and case studies,” J Intell Mater Syst Struct, vol. 30, no. 18–19, 2019, doi: 10.1177/1045389X19873390.
[26]. J. S. Koh, “Design of shape memory alloy coil spring actuator for improving performance in cyclic actuation,” Materials, vol. 11, no. 11, 2018, doi: 10.3390/ma11112324.
[27]. G. Scirè Mammano and E. Dragoni, “Elastic compensation of linear shape memory alloy actuators using compliant mechanisms,” in Journal of Intelligent Material Systems and Structures, 2014. doi: 10.1177/1045389X13488253.
[28]. S. Saito, S. Oka, and R. Onodera, “Modelling of a shape memory alloy actuator for feedforward hysteresis compensator considering load fluctuation,” CAAI Trans Intell Technol, vol. 7, no. 4, 2022, doi: 10.1049/cit2.12129.
[29]. E. A. Williams, G. Shaw, and M. Elahinia, “Control of an automotive shape memory alloy mirror actuator,” Mechatronics, vol. 20, no. 5, 2010, doi: 10.1016/j.mechatronics.2010.04.002.
[30]. H. F. M. Ali, Y. Kim, Q. H. Le, and B. Shin, “Modeling and control of two DOF shape memory alloy actuators with applications,” Microsystem Technologies, vol. 28, no. 10, 2022, doi: 10.1007/s00542-022-05301-z.
[31]. E. J. Abdullah, J. Soriano, I. Fernández de Bastida Garrido, and D. L. Abdul Majid, “Accurate position control of shape memory alloy actuation using displacement feedback and self-sensing system,” Microsystem Technologies, vol. 27, no. 7, 2021, doi: 10.1007/s00542-020-05085-0.
[32]. R. Neugebauer, K. Pagel, A. Bucht, and W. G. Drossel, “Model-based position control of Shape memory alloy actuators,” International Journal of Mechatronics and Manufacturing Systems, vol. 5, no. 2, 2012, doi: 10.1504/IJMMS.2012.046521.
[33]. A. Kumagai, T. I. Liu, and P. Hozian, “Control of shape memory alloy actuators with a neuro-fuzzy feedforward model element,” J Intell Manuf, vol. 17, no. 1, 2006, doi: 10.1007/s10845-005-5512-2.
[34]. N. T. Tai, N. B. Kha, and K. K. Ahn, “Predictive position and force control for shape memory alloy cylinders,” Journal of Mechanical Science and Technology, vol. 24, no. 8, 2010, doi: 10.1007/s12206-010-0504-3.
[35]. E. Shi, X. Zhong, T. Wang, X. Li, C. Bu, and X. Zhao, “Adaptive control for shape memory alloy actuated systems with applications to human–robot interaction,” Front Neurosci, vol. 18, 2024, doi: 10.3389/fnins.2024.1337580.
[36]. P. Senthilkumar, G. N. Dayananda, M. Umapathy, and V. Shankar, “Experimental evaluation of a shape memory alloy wire actuator with a modulated adaptive controller for position control,” Smart Mater Struct, vol. 21, no. 1, 2012, doi: 10.1088/0964-1726/21/1/015015.
[37]. H. Ashrafiuon and V. R. Jala, “Sliding mode control of mechanical systems actuated by shape memory alloy,” Journal of Dynamic Systems, Measurement and Control, Transactions of the ASME, vol. 131, no. 1, 2009, doi: 10.1115/1.3023121.
[38]. N. T. Tai and K. K. Ahn, “Adaptive proportional-integral-derivative tuning sliding mode control for a shape memory alloy actuator,” Smart Mater Struct, vol. 20, no. 5, 2011, doi: 10.1088/0964-1726/20/5/055010
Cite this article
Gao,R. (2024). Advancements in shape-memory alloys: Properties, applications, challenges, and future prospects. Applied and Computational Engineering,91,63-72.
Data availability
The datasets used and/or analyzed during the current study will be available from the authors upon reasonable request.
Disclaimer/Publisher's Note
The statements, opinions and data contained in all publications are solely those of the individual author(s) and contributor(s) and not of EWA Publishing and/or the editor(s). EWA Publishing and/or the editor(s) disclaim responsibility for any injury to people or property resulting from any ideas, methods, instructions or products referred to in the content.
About volume
Volume title: Proceedings of the 2nd International Conference on Functional Materials and Civil Engineering
© 2024 by the author(s). Licensee EWA Publishing, Oxford, UK. This article is an open access article distributed under the terms and
conditions of the Creative Commons Attribution (CC BY) license. Authors who
publish this series agree to the following terms:
1. Authors retain copyright and grant the series right of first publication with the work simultaneously licensed under a Creative Commons
Attribution License that allows others to share the work with an acknowledgment of the work's authorship and initial publication in this
series.
2. Authors are able to enter into separate, additional contractual arrangements for the non-exclusive distribution of the series's published
version of the work (e.g., post it to an institutional repository or publish it in a book), with an acknowledgment of its initial
publication in this series.
3. Authors are permitted and encouraged to post their work online (e.g., in institutional repositories or on their website) prior to and
during the submission process, as it can lead to productive exchanges, as well as earlier and greater citation of published work (See
Open access policy for details).