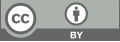
Research Progress on Metal-Based Materials in Photocatalytic CO2 Reduction
- 1 East China University of Science and Technology, No. 999 Hai Si Rd, Fengxian District, Shanghai, China
* Author to whom correspondence should be addressed.
Abstract
The photocatalytic reduction of CO2 into value-added chemicals is regarded as a vital approach to alleviate the environmental and energy crises. Photocatalytic technology is more energy-efficient and environmentally friendly compared to electrochemical, thermochemical, and other technologies, offering broad application prospects. Therefore, it is crucial to select high-performance photocatalytic materials. Metal compounds stand out in the field of photocatalysis due to their tunable band structures abundant active sites and excellent chemical stability. This review systematically summarizes the latest progress of metal-based materials, including metal oxides such as TiO2, CuO, Cu2O, WO3, as well as metal sulfides, metal phosphides, and metal-organic frameworks (MOFs), focusing on the mechanisms of strategies such as crystal engineering, heterojunction construction, and defect engineering in enhancing photocatalytic performance. Finally, it discusses the prospects and challenges of photocatalytic CO2 reduction technology, aiming to provide theoretical references for the development of ideal and efficient photocatalytic systems, contributing to the achievement of carbon neutrality goals.
Keywords
photocatalytic, CO2, carbon neutrality
[1]. World Meteorological Organization. (2024) WMO Confirms 2024 as Warmest Year on Record at About 1.55°C Above Pre-industrial Level. Retrieved from https://wmo.int/news/media-centre/wmo-confirms-2024-warmest-year-record-about-155degc-above-pre-industrial-level
[2]. Associated Press. (2024) Earth Breaks Yearly Heat Record and Lurches Past Dangerous Warming Threshold. AP News.Retrieved from https://apnews.com/article/climate-change-warming-hot-record-2024-disasters-12f899f071fcdbd051ad49a872611e92
[3]. National Aeronautics and Space Administration. (2024) Temperatures Rising: NASA Confirms 2024 Warmest Year on Record. Retrieved from https://www.nasa.gov/news-release/temperatures-rising-nasa-confirms-2024-warmest-year-on-record/
[4]. Mac Dowell, N., Fennell, P., Shah, N., et al. (2017) The Role of CO2 Capture and Utilization in Mitigating Climate Change. Nature Clim Change, 7, 243–249. https://doi.org/10.1038/nclimate3231
[5]. Hepburn, C., Adlen, E., Beddington, J., et al. (2019) The Technological and Economic Prospects for CO2 Utilization and Removal. Nature, 575, 87–97. https://doi.org/10.1038/s41586-019-1681-6
[6]. Hepburn, C., Adlen, E., Beddington, J., et al. (2019) The Technological and Economic Prospects for CO2 Utilization and Removal. Nature, 575, 87–97. https://doi.org/10.1038/s41586-019-1681-6
[7]. Li, D., et al. (2024) Strategies for Optimizing the Efficiency and Selectivity of Photocatalytic Aqueous CO2 Reduction: Catalyst Design and Operating Conditions. Nano Energy, 110460.
[8]. Liu, B., et al. (2017) Phosphorus-Doped Graphitic Carbon Nitride Nanotubes with Amino-Rich Surface for Efficient CO2 Capture, Enhanced Photocatalytic Activity and Product Selectivity. ACS Appl Mater Interfaces. DOI: 10.1021/acsami.7b17503
[9]. Bafaqeer, A., Tahir, M., and Amin, N.A.S. (2019) Well-Designed ZnV2O6/g-C3N4 2D/2D Nanosheets Heterojunction with Faster Charges Separation via pCN as Mediator Towards Enhanced Photocatalytic Reduction of CO2 to Fuels. Applied Catalysis B: Environmental, 242, 312–326.
[10]. Zhao, L., et al. (2023) Efficient Photoreduction of Carbon Dioxide into Carbon-Based Fuels: A Review. Environmental Chemistry Letters, 21, 1499–1513.
[11]. Gao, Y., et al. (2020) Recent Advances in Visible-Light-Driven Conversion of CO2 by Photocatalysts into Fuels or Value-Added Chemicals. Carbon Resources Conversion, 3, 46–59.
[12]. Zhao, S., et al. (2012) g-C3N4/TiO2 Hybrid Photocatalyst with Wide Absorption Wavelength Range and Effective Photogenerated Charge Separation. Separation and Purification Technology, 99, 50–54.
[13]. Gong, et al. (2016) CO2 Photo-Reduction: Insights into CO2 Activation and Reaction on Surfaces of Photocatalysts. Energy & Environmental Science.
[14]. Tachibana, Y., Vayssieres, L., and Durrant, J.R. (2012) Artificial Photosynthesis for Solar Water-Splitting. Nature Photonics, 6, 511–518.
[15]. Noue, T., Fujishima, A., Konishi, S., et al. (1979) Photoelectrocatalytic Reduction of Carbon Dioxide in Aqueous Suspensions of Semiconductor Powders. Nature, 277, 637–638. https://doi.org/10.1038/277637a0
[16]. Yuan, Z., et al. Enhancing Photocatalytic CO2 Reduction with TiO2-Based Materials: Strategies, Mechanisms, Challenges, and Perspectives. Environmental Science and Ecotechnology, 20, 100368.
[17]. Wang, S., et al. (2021) Mechanistic Insight into Photocatalytic CO2 Reduction by a Z-Scheme gC3N4/TiO2 Heterostructure. New Journal of Chemistry, 45, 11474–11480.
[18]. Zhou, Y., et al. (2022) Photocatalytic Reduction of CO2 into CH4 over Ru-Doped TiO2: Synergy of Ru and Oxygen Vacancies. Journal of Colloid and Interface Science, 608, 2809–2819.
[19]. Kmentová, H., et al. (2025) Tuning CO2 Reduction Selectivity via Structural Doping of TiO2 Photocatalysts. Journal of CO2 Utilization, 91, 103008.
[20]. Asahi, R., et al. (2001) Visible-Light Photocatalysis in Nitrogen-Doped Titanium Oxides. Science, 293, 269–271.
[21]. Khlyustova, A., et al. (2020) Doped TiO2: The Effect of Doping Elements on Photocatalytic Activity. Materials Advances, 1.
[22]. Di, T., Zhang, J., Cheng, B., et al. (2018) Hierarchically Nanostructured Porous TiO2(B) with Superior Photocatalytic CO2 Reduction Activity. Science China Chemistry, 61, 344–350. https://doi.org/10.1007/s11426-017-9174-9
[23]. Bai, S., Wang, L., Li, Z. and Xiong Y.Z. (2017) Facet-Engineered Surface and Interface Design of Photocatalytic Materials. Advanced Science. Advanced Science, 4(1), 1600216.
[24]. Reñones, P., et al. (2016) Hierarchical TiO2 Nanofibres as Photocatalyst for CO2 Reduction: Influence of Morphology and Phase Composition on Catalytic Activity. Journal of CO2 Utilization, 15, 24–31.
[25]. Dehkordi, A.B., et al. (2020) Preparation of Hierarchical g-C3N4@TiO2 Hollow Spheres for Enhanced Visible-Light Induced Catalytic CO2 Reduction. Solar Energy, 205, 465–473.
[26]. Chen, S., et al. (2018) In-Situ Growth of ZnIn2S4 Decorated on Electrospun TiO2 Nanofibers with Enhanced Visible-Light Photocatalytic Activity. Chemical Physics Letters, 706, 68–75.
[27]. Wang, J., et al. (2022) A Review on TiO2−x-Based Materials for Photocatalytic CO2 Reduction. Nanoscale, 14, 11512–11528.
[28]. Zhang, W., et al. (2021) Black Single-Crystal TiO2 Nanosheet Array Films with Oxygen Vacancy on {001} Facets for Boosting Photocatalytic CO2 Reduction. Journal of Alloys and Compounds, 870, 159400.
[29]. Huang, S., Wang, J.S., Bao, R., Yi, J.H., Liu, L., Kong, X.(2024) Oxygen Vacancy-Induced Modulation of the Built-in Electric Field of TiO2-α/WO3-δ for Enhanced CO2 Photoreduction. Ceramics International, 50, 38323–38330. https://doi.org/10.1016/j.ceramint.2024.07.196
[30]. Li, Y., Ren, Z., and Gu, M. (2022) Synergistic Effect of Interstitial C Doping and Oxygen Vacancies on the Photoreactivity of TiO2 Nanofibers Towards CO2 Reduction. Applied Catalysis B: Environmental.
[31]. Li, Z., et al. (2022) Engineered Disorder in CO2 Photocatalysis. Nature Communications, 13, 7205.
[32]. Zhang, J., Xu, J., and Tao, F. (2021) Interface Modification of TiO2 Nanotubes by Biomass-Derived Carbon Quantum Dots for Enhanced Photocatalytic Reduction of CO2. ACS Applied Energy Materials, 4, 13120–13131.
[33]. Liu, E., et al. (2014) Photocatalytic Reduction of CO2 into Methanol over Ag/TiO2 Nanocomposites Enhanced by Surface Plasmon Resonance. Plasmonics, 9, 61–70.
[34]. Xing, M., et al. (2018) Modulation of the Reduction Potential of TiO2-x by Fluorination for Efficient and Selective CH4 Generation from CO2 Photoreduction. Nano Letters. DOI: 10.1021/acs.nanolett.8b00197
[35]. Li, J.Y., et al. (2019) One-Dimensional Copper-Based Heterostructures Toward Photo-Driven Reduction of CO2 to Sustainable Fuels and Feedstocks. Journal of Materials Chemistry A, 7, 8676–8689.
[36]. Zhang, X., et al. (2021) Palladium-Modified Cuprous(I) Oxide with {100} Facets for Photocatalytic CO2 Reduction. Nanoscale, 13.
[37]. Xue, J., et al. (2022) Three-Dimensional Porous Cu2O with Dendrite for Efficient Photocatalytic Reduction of CO2 Under Visible Light. Applied Surface Science, 581.
[38]. Gusain, R., et al. (2016) Reduced Graphene Oxide-CuO Nanocomposites for Photocatalytic Conversion of CO2 into Methanol Under Visible Light Irradiation. Applied Catalysis B: Environmental, 352–362.
[39]. Wang, Z., et al. (2024) Constructing Cuprous Oxide-Modified Zinc Tetraphenylporphyrin Ultrathin Nanosheets Heterojunction for Enhanced Photocatalytic Carbon Dioxide Reduction to Methane. Journal of Colloid and Interface Science, 667, 212–222.
[40]. Huang, G., et al. (2022) Unique Insights into Photocatalytic VOCs Oxidation Over WO3/Carbon Dots Nanohybrids Assisted by Water Activation and Electron Transfer at Interfaces. Journal of Hazardous Materials, 423, 127134.
[41]. Liu, Z., Wu, J., and Zhang, J. (2016) Quantum Dots and Plasmonic Ag Decorated WO3 Nanorod Photoanodes with Enhanced Photoelectrochemical Performances. International Journal of Hydrogen Energy, 41, 20529–20535.
[42]. Shi, W., et al. (2019) Controllable Synthesis of Cu2O Decorated WO3 Nanosheets with Dominant (001) Facets for Photocatalytic CO2 Reduction Under Visible-Light Irradiation. Applied Catalysis B: Environmental, 243, 236–242.
[43]. Gao, W., et al. (2020) Anchoring of Black Phosphorus Quantum Dots onto WO3 Nanowires to Boost Photocatalytic CO2 Conversion into Solar Fuels. Chemical Communications, 56, 7777–7780.
[44]. Zhu, B., et al. (2022) Enhanced Photocatalytic CO2 Reduction Over 2D/1D BiOBr0.5Cl0.5/WO3 S-Scheme Heterostructure. Acta Physico-Chimica Sinica, 38, 2111008.
[45]. Wang, H., et al. (2020) Photocatalytic CO2 Reduction Over Platinum Modified Hexagonal Tungsten Oxide: Effects of Platinum on Forward and Back Reactions. Applied Catalysis B: Environmental, 263.
[46]. Lei, B., et al. (2022) C-Doped Induced Oxygen-Vacancy in WO3 Nanosheets for CO2 Activation and Photoreduction. ACS Catalysis, 12, 9670–9678.
[47]. Zeng, D., et al. (2023) Photocatalytic Conversion of CO2 to Acetic Acid by CuPt/WO3: Chloride Enhanced CC Coupling Mechanism. Applied Catalysis B: Environmental, 323, 122177.
[48]. Chen, Y., et al. (2017) Metal–Organic Frameworks (MOFs) for Photocatalytic CO2 Reduction. Catalysis Science & Technology, 7, 4893–4904.
[49]. Liu, Y., et al. (2024) Recent Advances in Amino-Functionalized Metal–Organic Frameworks for Sustainable Photocatalytic Carbon Dioxide Reduction. Separation and Purification Technology, 131023.
[50]. Gu, L., et al. (2022) Optimization of Fe/Ni Organic Frameworks with Core–Shell Structures for Efficient Visible-Light-Driven Reduction of Carbon Dioxide to Carbon Monoxide. Nanoscale, 14, 15821–15831.
[51]. Cheng, X.M., Zhang, X.Y., Dao, X.Y., et al. (2022) High-Index Facets Exposed on Metal–Organic Framework for Boosting Photocatalytic Carbon Dioxide Reduction. Chemical Engineering Journal, 431, 134125.
[52]. Shen, L., et al. (2015) Electronic Effects of Ligand Substitution on Metal–Organic Framework Photocatalysts: The Case Study of UiO-66. Physical Chemistry Chemical Physics, 17, 117–121.
[53]. Huang, A., Wan, L., and Caro, J. (2018) Microwave-Assisted Synthesis of Well-Shaped UiO-66-NH2 with High CO2 Adsorption Capacity. Materials Research Bulletin, 98, 308–313.
[54]. Cheng, L., et al. (2021) Structural Engineering of 3D Hierarchical Cd0.8Zn0.2S for Selective Photocatalytic CO2 Reduction. Chinese Journal of Catalysis, 42, 131–140.
[55]. Xu, Y., et al. (2022) In-Situ Growth of Metal Phosphide-Black Phosphorus Heterojunction for Highly Selective and Efficient Photocatalytic Carbon Dioxide Conversion. Journal of Colloid and Interface Science, 616, 641–648.
[56]. Zhao, L., et al. (2023) Efficient Photoreduction of Carbon Dioxide into Carbon-Based Fuels: A Review. Environmental Chemistry Letters, 21, 1499–1513.
Cite this article
Song,J. (2025). Research Progress on Metal-Based Materials in Photocatalytic CO2 Reduction. Applied and Computational Engineering,149,60-70.
Data availability
The datasets used and/or analyzed during the current study will be available from the authors upon reasonable request.
Disclaimer/Publisher's Note
The statements, opinions and data contained in all publications are solely those of the individual author(s) and contributor(s) and not of EWA Publishing and/or the editor(s). EWA Publishing and/or the editor(s) disclaim responsibility for any injury to people or property resulting from any ideas, methods, instructions or products referred to in the content.
About volume
Volume title: Proceedings of CONF-MSS 2025 Symposium: Automation and Smart Technologies in Petroleum Engineering
© 2024 by the author(s). Licensee EWA Publishing, Oxford, UK. This article is an open access article distributed under the terms and
conditions of the Creative Commons Attribution (CC BY) license. Authors who
publish this series agree to the following terms:
1. Authors retain copyright and grant the series right of first publication with the work simultaneously licensed under a Creative Commons
Attribution License that allows others to share the work with an acknowledgment of the work's authorship and initial publication in this
series.
2. Authors are able to enter into separate, additional contractual arrangements for the non-exclusive distribution of the series's published
version of the work (e.g., post it to an institutional repository or publish it in a book), with an acknowledgment of its initial
publication in this series.
3. Authors are permitted and encouraged to post their work online (e.g., in institutional repositories or on their website) prior to and
during the submission process, as it can lead to productive exchanges, as well as earlier and greater citation of published work (See
Open access policy for details).