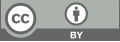
Status and prospects of wide bandgap semiconductor devices
- 1 Beijing Institute of Technology
- 2 Beijing Institute of Technology
* Author to whom correspondence should be addressed.
Abstract
Wide bandgap semiconductor materials, such as silicon carbide (SiC) and gallium nitride (GaN), have attracted significant attention due to their exceptional electronic and thermal properties, making them ideal for high-power and high-frequency applications. This study provides a comprehensive review of SiC and GaN materials and recent developments in power electronics, radio frequency (RF) devices. The study focuses on the unique properties of these materials, which enable them to outperform traditional silicon-based devices in terms of efficiency, power density, and overall performance. The objectives include examining material properties, assessing SiC and GaN-based device performance, comparing electron mobility, on-resistance, and temperature dependence, and identifying areas for future research. The content covers a range of devices, including SiC MOSFETs, IGBTs, GaN HEMTs, and their applications, with a focus on recent advances in material quality and device reliability. The research contribution of the paper lies in its comprehensive analysis, which serves as a valuable reference for researchers, manufacturers, and policymakers working to accelerate the development of advanced materials and technologies. The successful implementation of SiC and GaN-based devices will lead to more energy-efficient systems, enhanced performance across various sectors, and a reduction in global energy consumption and environmental impact, revolutionizing the electronics industry.
Keywords
wide bandgap semiconductor devices, SiC, GaN, power electronics
[1]. Han L., Liang L., Kang Y., Qiu Y., 2021, A Review of SiC IGBT: Models, Fabrications, Characteristics, and Applications, IEEE Transactions on Power Electronics 36(2) 2080-2093.
[2]. Chaujar R., 2019, Analog and RF assessment of sub-20 nm 4H-SiC trench gate MOSFET for high frequency applications, AEU - International Journal of Electronics and Communications 98 51-57.
[3]. Pushpakaran B. N., Subburaj A. S., Bayne S. B., 2020, Commercial GaN-Based Power Electronic Systems: A Review, Journal of Electronic Materials 49(11) 6247-6262.
[4]. Zhang Y., Zubair A., Liu Z., Xiao M., Perozek J., Ma Y., Palacios T., 2021, GaN FinFETs and trigate devices for power and RF applications: Review and perspective, Semiconductor Science and Technology 36(5) 054001.
[5]. Duffy M. R., Lasser G., Nevett G., Roberg M., Popović Z., 2019, A three-stage 18.5–24-GHz GaN-on-SiC 4 W 40% efficient MMIC PA, IEEE Journal of Solid-State Circuits 54(9) 2402-2410.
[6]. El-Helou A., Cui Y., Tadjer M., Anderson T., Francis D., Feygelson T., Pate B., Hobart K., Raad P., 2020, Full thermal characterization of AlGaN/GaN high electron mobility transistors on silicon, silicon carbide, and diamond substrates using a reverse modeling approach, Semiconductor Science and Technology 36(1) 014008.
[7]. Fisicaro G., Bongiorno C., Deretzis I., Giannazzo F., Via F. L., Roccaforte F., Zielinski M., Zimbone M., Magna A. L., 2020, Genesis and evolution of extended defects: The role of evolving interface instabilities in cubic SiC, Applied Physics Reviews 7(2) 021402.
[8]. Kawaguchi K., Wang Y., Xu J., Ootani Y., Higuchi Y., Ozawa N., Kubo M., 2021, Cooperative roles of chemical reactions and mechanical friction in chemical mechanical polishing of gallium nitride assisted by OH radicals: tight-binding quantum chemical molecular dynamics simulations, Physical Chemistry Chemical Physics 23(7) 4075-4084.
[9]. Pu T., Younis U., Chiu H.-C., Xu K., Kuo H.-C., Liu X., 2021, Review of Recent Progress on Vertical GaN-Based PN Diodes, Nanoscale Research Letters 16(1) 101.
[10]. Tian W., Wu Y., Wu T., Dou L., Cao X., Li J., 2022, Mechanisms and Performance Analysis of GaN-Based Micro-LED Grown on Pattern Sapphire Substrate by Laser Lift-Off Process, ECS Journal of Solid State Science and Technology 11(4) 046001.
[11]. Peng Z., 2021, Design and experimental study of 1200V 4H-SiC planar MOSFET, University of Electronic Science and Technology.
[12]. Zhu S., Liu T., Fan J., Salemi A., White M. H., Sheridan D., Agarwal A. K., 2022, A New Cell Topology for 4H-SiC Planar Power MOSFETs for High-Frequency Switching, Materials 15(19) 6690.
[13]. Yu H., Wang J., Deng G., Liang S., Liu H., Shen Z. J., 2022, A Novel 4H-SiC JBS-Integrated MOSFET With Self-Pinching Structure for Improved Short-Circuit Capability, IEEE Transactions on Electron Devices 69(9) 5104-5109.
[14]. Xu X., 2021, Study on novel structures for 1.2kV SiC MOSFETs, University of Electronic Science and Technology.
[15]. Tang L., 2020, Design of 1.2kV trench SiC power MOSFET, Southeast University.
[16]. Yang W., 2019, New structure design and characteristics research of SiC UMOSFET, University of Electronic Science and Technology.
[17]. Vudumula P., Kotamraju S., 2019, Design and Optimization of SiC Super-Junction MOSFET Using Vertical Variation Doping Profile, IEEE Transactions on Electron Devices 66(3) 1402-1408.
[18]. Cao W., Li M., Ou Y., Liu D., 2022, 1.2-kV SiC Superjunction Dual-channel MOSFET with Ultra Low Specific on-Resistance, 2022 IEEE 17th Conference on Industrial Electronics and Applications (ICIEA), pp. 64-68.
[19]. Das S., Zheng Y., Ahyi A., Kuroda M. A., Dhar S., 2022, Study of Carrier Mobilities in 4H-SiC MOSFETS Using Hall Analysis, Materials 15(19) 6736.
[20]. Arith F., Urresti J., Vasilevskiy K., Olsen S., Wright N., O’Neill A., 2018, Increased Mobility in Enhancement Mode 4H-SiC MOSFET Using a Thin SiO2 / Al2O3 Gate Stack, IEEE Electron Device Letters 39(4) 564-567.
[21]. Li F., Sharma Y. K., Jennings M. R., Pérez-Tomás A., Shah V. A., Rong H., Russell S., Martin D. M., Mawby P. A., 2016, Improved Channel Mobility by Oxide Nitridation for N-Channel MOSFET on 3C-SiC(100)/Si, Materials Science Forum 858 667-670.
[22]. Noguchi M., Watanabe T., Watanabe H., Kita K., Miura N., 2021, Comparative Study of Hall Effect Mobility in Inversion Layer of 4H-SiC MOSFETs With Nitrided and Phosphorus-Doped Gate Oxides, IEEE Transactions on Electron Devices 68(12) 6321-6329.
[23]. Hong K., Chen X. Y., Chen Y., Zhang M. S., Wang J. L., Jiang S., Pang Z., Yang H. M., Xue N., Gou H. Y., Zeng L., 2019, Experimental Investigations Into Temperature and Current Dependent On-State Resistance Behaviors of 1.2 kV SiC MOSFETs, IEEE Journal of the Electron Devices Society 7 925-930.
[24]. Sun J., Xu H., Wu X., Yang S., Guo Q., Sheng K., 2017, Short circuit capability and high temperature channel mobility of SiC MOSFETs, 2017 29th International Symposium on Power Semiconductor Devices and IC's (ISPSD), pp. 399-402.
[25]. Moscatelli F., Poggi A., Solmi S., Nipoti R., 2008, Nitrogen Implantation to Improve Electron Channel Mobility in 4H-SiC MOSFET, IEEE Transactions on Electron Devices 55(4) 961-967.
[26]. Liu T., Zhu S., Salemi A., Sheridan D., White M. H., Agarwal A. K., 2021, JFET Region Design Trade-Offs of 650 V 4H-SiC Planar Power MOSFETs, Solid State Electronics Letters 3 53-58.
[27]. Shrestha N., Mazumder S. K., Voss L., 2021, Electrical Properties of Optically Triggered SiC JFET for Power Electronic Application, 2021 IEEE 12th International Symposium on Power Electronics for Distributed Generation Systems (PEDG), pp. 1-6.
[28]. Funaki T., Kashyap A. S., Mantooth H. A., Balda J. C., Barlow F. D., Kimoto T., Hikihara T., 2006, Characterization of SiC JFET for temperature dependent device modeling, 2006 37th IEEE Power Electronics Specialists Conference, pp. 1-6.
[29]. Wen Z.-X., Zhang F., Shen Z.-W., Chen J., He Y.-W., Yan G.-G., Liu X.-F., Zhao W.-S., Wang L., Sun G.-S., Zeng Y.-P., 2019, Design and fabrication of 10-kV silicon–carbide p-channel IGBTs with hexagonal cells and step space modulated junction termination extension*, Chinese Physics B 28(6) 068504.
[30]. Watanabe N., Okino H., Shima A., 2022, Impact of Cell Layout on On-state and Dynamic Characteristics of N-channel SiC IGBTs, 2022 IEEE 34th International Symposium on Power Semiconductor Devices and ICs (ISPSD), pp. 85-88.
[31]. Deng X., Cheng Z., Chen Z., Wu H., Bai S., Li X., Li X., Chen W., Zhang B., 2022, A Hybrid-Channel Injection Enhanced Modulation 4H-SiC IGBT Transistors With Improved Performance, IEEE Transactions on Electron Devices 69(8) 4421-4426.
[32]. Matsuura K., Tanimoto Y., Saito A., Miyaoku Y., Mizoguchi T., Miura-Mattausch M., Mattausch H. J., 2019, Analysis and compact modeling of temperature-dependent switching in SiC IGBT circuits, Solid-State Electronics 153 59-66.
[33]. Mishra U. K., Parikh P., Wu Y.-F., 2002, AlGaN/GaN HEMTs-an overview of device operation and applications, Proceedings of the IEEE 90(6) 1022-1031.
[34]. Sequeira M. C., Mattei J.-G., Vazquez H., Djurabekova F., Nordlund K., Monnet I., Mota-Santiago P., Kluth P., Grygiel C., Zhang S., Alves E., Lorenz K., 2021, Unravelling the secrets of the resistance of GaN to strongly ionising radiation, Communications Physics 4(1) 51.
[35]. Pérez-Tomás A., Fontserè A., 2011, AlGaN/GaN hybrid MOS-HEMT analytical mobility model, Solid-State Electronics 56(1) 201-206.
[36]. Smith M., Kajiwara Y., Ono H., Huang P. C., Kato D., Mukai A., Shindome A., Kuraguchi M., 2021, High mobility in GaN MOSFETs with AlSiO gate dielectric and AlN mobility enhancement layer, 2021 IEEE 8th Workshop on Wide Bandgap Power Devices and Applications (WiPDA), pp. 283-287.
[37]. Poncé S., Jena D., Giustino F., 2019, Hole mobility of strained GaN from first principles, Physical Review B 100(8) 085204.
[38]. Li S., Liu S., Tian Y., Zhang C., Wei J., Tao X., Li N., Zhang L., Sun W., 2020, High-temperature electrical performances and physics-based analysis of p-GaN HEMT device, IET Power Electronics 13(3) 420-425.
[39]. Mohanty S. K., Chen Y.-Y., Yeh P.-H., Horng R.-H., 2019, Thermal Management of GaN-on-Si High Electron Mobility Transistor by Copper Filled Micro-Trench Structure, Scientific Reports 9(1) 19691.
Cite this article
Zhang,M.;Zhang,Y. (2023). Status and prospects of wide bandgap semiconductor devices. Applied and Computational Engineering,23,252-262.
Data availability
The datasets used and/or analyzed during the current study will be available from the authors upon reasonable request.
Disclaimer/Publisher's Note
The statements, opinions and data contained in all publications are solely those of the individual author(s) and contributor(s) and not of EWA Publishing and/or the editor(s). EWA Publishing and/or the editor(s) disclaim responsibility for any injury to people or property resulting from any ideas, methods, instructions or products referred to in the content.
About volume
Volume title: Proceedings of the 2023 International Conference on Functional Materials and Civil Engineering
© 2024 by the author(s). Licensee EWA Publishing, Oxford, UK. This article is an open access article distributed under the terms and
conditions of the Creative Commons Attribution (CC BY) license. Authors who
publish this series agree to the following terms:
1. Authors retain copyright and grant the series right of first publication with the work simultaneously licensed under a Creative Commons
Attribution License that allows others to share the work with an acknowledgment of the work's authorship and initial publication in this
series.
2. Authors are able to enter into separate, additional contractual arrangements for the non-exclusive distribution of the series's published
version of the work (e.g., post it to an institutional repository or publish it in a book), with an acknowledgment of its initial
publication in this series.
3. Authors are permitted and encouraged to post their work online (e.g., in institutional repositories or on their website) prior to and
during the submission process, as it can lead to productive exchanges, as well as earlier and greater citation of published work (See
Open access policy for details).