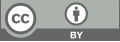
A promising chalcogenide perovskite-BaZrS3-for application on tandem solar cells
- 1 Department of chemical engineering, University of Florida, Gainesville, FL 32603
* Author to whom correspondence should be addressed.
Abstract
Applying a tandem structure to silicon-based sub-cells is the most promising strategy for enhancing efficiency and overcoming the Shockley-Queisser limit, which is an intrinsic limitation of silicon-based solar cells. This study will begin with the various categories of tandem solar cells and then analyze the requirements for optimizing the overall conversion efficiency. Therefore, the characteristics of BaZrS3, a chalcogenide perovskite with high elemental abundance, exceptional photovoltaic properties as well as outstanding stability, are thoroughly evaluated and compared with other previously utilized materials for the top sub-cell. Finally, the most feasible approach for thin film growth which is physical vapor deposition is reviewed based on the available literature from bulk synthesis of BaZrS3. This evaluation may serve as a critical guideline for the future commercialization of BaZrS3.
Keywords
chalcogenide perovskite, tandem solar cell, solar energy
[1]. Yoshikawa K, Kawasaki H, Yoshida W, Irie T, Konishi K, Nakano K, Uto T, Adachi D, Kanematsu M, Uzu H, Yamamoto K. Silicon heterojunction solar cell with interdigitated back contacts for a photoconversion efficiency over 26%. Nature energy. 2017 Mar 20;2(5):1-8.
[2]. Shockley W, Queisser HJ. Detailed balance limit of efficiency of p‐n junction solar cells. Journal of applied physics. 1961 Mar;32(3):510-9.
[3]. Cariou R, Benick J, Feldmann F, Höhn O, Hauser H, Beutel P, Razek N, Wimplinger M, Bläsi B, Lackner D, Hermle M. III–V-on-silicon solar cells reaching 33% photoconversion efficiency in two-terminal configuration. Nature Energy. 2018 Apr;3(4):326-33.
[4]. Essig S, Allebé C, Remo T, Geisz JF, Steiner MA, Horowitz K, Barraud L, Ward JS, Schnabel M, Descoeudres A, Young DL. Raising the one-sun conversion efficiency of III–V/Si solar cells to 32.8% for two junctions and 35.9% for three junctions. Nature Energy. 2017 Aug 25;2(9):1-9.
[5]. Minami T. Transparent conducting oxide semiconductors for transparent electrodes. Semiconductor science and technology. 2005 Mar 15;20(4):S35.
[6]. De Vos A. Detailed balance limit of the efficiency of tandem solar cells. Journal of Physics D: Applied Physics. 1980 May 14;13(5):839.
[7]. Dubey A, Adhikari N, Mabrouk S, Wu F, Chen K, Yang S, Qiao Q. A strategic review on processing routes towards highly efficient perovskite solar cells. Journal of Materials Chemistry A. 2018;6(6):2406-31.
[8]. Xie H, Lira-Cantu M. Multi-component engineering to enable long-term operational stability of perovskite solar cells. Journal of Physics: Energy. 2020 Apr 24;2(2):024008.
[9]. M. Fischer, M. Woodhouse, S. Herritsch, J. Trube, International Technology Roadmap for Photovoltaic (ITRPV), VDMA E. V. Photovoltaic Equipment, Frankfurt, Germany 2020.
[10]. Liu J, De Bastiani M, Aydin E, Harrison GT, Gao Y, Pradhan RR, Eswaran MK, Mandal M, Yan W, Seitkhan A, Babics M. Efficient and stable perovskite-silicon tandem solar cells through contact displacement by MgF x. Science. 2022 Jun 23:eabn8910.
[11]. Jain A, Ong SP, Hautier G, Chen W, Richards WD, Dacek S, Cholia S, Gunter D, Skinner D, Ceder G, Persson KA. Commentary: The Materials Project: A materials genome approach to accelerating materials innovation. APL materials. 2013 Jul 18;1(1):011002.
[12]. Meng W, Saparov B, Hong F, Wang J, Mitzi DB, Yan Y. Alloying and defect control within chalcogenide perovskites for optimized photovoltaic application. Chemistry of Materials. 2016 Feb 9;28(3):821-9.
[13]. Gupta T, Ghoshal D, Yoshimura A, Basu S, Chow PK, Lakhnot AS, Pandey J, Warrender JM, Efstathiadis H, Soni A, Osei‐Agyemang E. An Environmentally Stable and Lead‐Free Chalcogenide Perovskite. Advanced Functional Materials. 2020 Jun;30(23):2001387.
[14]. Wei X, Hui H, Zhao C, Deng C, Han M, Yu Z, Sheng A, Roy P, Chen A, Lin J, Watson DF. Realization of BaZrS3 chalcogenide perovskite thin films for optoelectronics. Nano Energy. 2020 Feb 1;68:104317.
[15]. Zeng H. Green, stable and earth abundant ionic PV absorbers based on chalcogenide perovskite. State Univ. of New York (SUNY), Univ. at Buffalo, Buffalo, NY (United States); 2018 May 16.
[16]. Ross RT. Some thermodynamics of photochemical systems. The Journal of Chemical Physics. 1967 Jun 15;46(12):4590-3.
[17]. Cabas‐Vidani A, Haass SG, Andres C, Caballero R, Figi R, Schreiner C, Márquez JA, Hages C, Unold T, Bleiner D, Tiwari AN. High‐Efficiency (LixCu1− x) 2ZnSn (S, Se) 4 Kesterite Solar Cells with Lithium Alloying. Advanced Energy Materials. 2018 Dec;8(34):1801191.
[18]. Giorgi G, Fujisawa JI, Segawa H, Yamashita K. Small photocarrier effective masses featuring ambipolar transport in methylammonium lead iodide perovskite: a density functional analysis. The journal of physical chemistry letters. 2013 Dec 19;4(24):4213-6.
[19]. Herz LM. Charge-carrier mobilities in metal halide perovskites: fundamental mechanisms and limits. ACS Energy Letters. 2017 Jul 14;2(7):1539-48.
[20]. Chen S, Walsh A, Gong XG, Wei SH. Classification of lattice defects in the kesterite Cu2ZnSnS4 and Cu2ZnSnSe4 earth‐abundant solar cell absorbers. Advanced materials. 2013 Mar 20;25(11):1522-39.
[21]. Wu X, Gao W, Chai J, Ming C, Chen M, Zeng H, Zhang P, Zhang S, Sun YY. Defect tolerance in chalcogenide perovskite photovoltaic material BaZrS3. Science China Materials. 2021 Dec;64(12):2976-86.
[22]. Jackson AJ, Tiana D, Walsh A. A universal chemical potential for sulfur vapours. Chemical science. 2016;7(2):1082-92.
[23]. Manser JS, Saidaminov MI, Christians JA, Bakr OM, Kamat PV. Making and breaking of lead halide perovskites. Accounts of chemical research. 2016 Feb 16;49(2):330-8.
Cite this article
Qin,S. (2023). A promising chalcogenide perovskite-BaZrS3-for application on tandem solar cells. Applied and Computational Engineering,7,14-23.
Data availability
The datasets used and/or analyzed during the current study will be available from the authors upon reasonable request.
Disclaimer/Publisher's Note
The statements, opinions and data contained in all publications are solely those of the individual author(s) and contributor(s) and not of EWA Publishing and/or the editor(s). EWA Publishing and/or the editor(s) disclaim responsibility for any injury to people or property resulting from any ideas, methods, instructions or products referred to in the content.
About volume
Volume title: Proceedings of the 3rd International Conference on Materials Chemistry and Environmental Engineering (CONF-MCEE 2023), Part II
© 2024 by the author(s). Licensee EWA Publishing, Oxford, UK. This article is an open access article distributed under the terms and
conditions of the Creative Commons Attribution (CC BY) license. Authors who
publish this series agree to the following terms:
1. Authors retain copyright and grant the series right of first publication with the work simultaneously licensed under a Creative Commons
Attribution License that allows others to share the work with an acknowledgment of the work's authorship and initial publication in this
series.
2. Authors are able to enter into separate, additional contractual arrangements for the non-exclusive distribution of the series's published
version of the work (e.g., post it to an institutional repository or publish it in a book), with an acknowledgment of its initial
publication in this series.
3. Authors are permitted and encouraged to post their work online (e.g., in institutional repositories or on their website) prior to and
during the submission process, as it can lead to productive exchanges, as well as earlier and greater citation of published work (See
Open access policy for details).