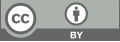
Traditional and Innovative Battery Recycling Methods
- 1 Indian Springs School, Pelham, AL 35124 USA
* Author to whom correspondence should be addressed.
Abstract
With the rapid increase in global demand for high-performance batteries, particularly driven by the growth of electric vehicles and large-scale clean energy storage systems, the need for effective battery recycling methods has become more pressing than ever. This paper provides a comprehensive examination of both traditional and innovative methods for battery recycling, which has become increasingly critical due to the growing environmental concerns associated with the disposal of batteries, particularly from electric vehicles and large-scale energy storage systems. The study analyzes three primary recycling methods: mechanical processing, chemical recovery, and direct reuse, detailing their underlying principles, advantages, and limitations. In addition, the paper introduces an innovative recycling approach that integrates bioremediation microorganisms and nanotechnology to enhance the efficiency of metal recovery and minimize environmental impact. This novel method offers a pollution-free, sustainable solution that addresses the inefficiencies and environmental risks associated with traditional recycling techniques. Through a comparative analysis, the study highlights the potential of this innovative approach to revolutionize battery recycling, contributing to more sustainable practices and the circular economy. The findings emphasize the importance of adopting advanced recycling technologies to meet the increasing demand for battery materials while ensuring environmental protection.
Keywords
Battery Recycling, Mechanical Processing, Chemical Recovery, Direct Reuse, Nanotechnology.
[1]. Marcott, S. A., Shakun, J. D., Clark, P. U. and Mix, A. C. (2013) A Reconstruction of Regional and Global Temperature for the Past 11,300 Years. Science, 339(6124), 1198–1201. doi:10.1126/science.1228026
[2]. Meredith, M., M. Sommerkorn, S. Cassotta, C. Derksen, A. Ekaykin, A. Hollowed, G. Kofinas, A. Mackintosh, J. Melbourne-Thomas, M.M.C. Muelbert, G. Ottersen, H. Pritchard, and E.A.G. Schuur, (2019) Polar Regions. In: IPCC Special Report on the Ocean and Cryosphere in a Changing Climate [H.-O. Pörtner, D.C. Roberts, V. Masson-Delmotte, P. Zhai, M. Tignor, E. Poloczanska, K. Mintenbeck, A. Alegría, M. Nicolai, A. Okem, J. Petzold, B. Rama, N.M. Weyer (eds.)]. In press.
[3]. Kheirinik, M., Ahmed, S. and Rahmanian, N. (2021) Comparative Techno-Economic Analysis of Carbon Capture Processes: Pre-Combustion, Post-Combustion, and Oxyfuel Combustion Operations. Sustainability, 13(24), 13567. doi:10.3390/su132413567
[4]. Yan, B., et al. (2016) Sustained deposition of contaminants from the Deepwater Horizon spill. Proceedings of the National Academy of Sciences, 113(15), E2073-E2082. doi:10.1073/pnas.1513155113
[5]. Manoj, B. and Ghosh, S. K. (2019) Current research and development trends of plastic waste recycling: A review. Waste Management, 84, 71-77. doi:10.1016/j.wasman.2018.12.034
[6]. Zhang, X., Wang, L., Zhang, Y. and Wang, S. (2020) Nanomaterials in environmental protection applications: A review on synthesis, performance, and challenges. Environmental Science: Nano, 7(7), 1822-1851. doi:10.1039/D0EN00123J
[7]. Zeng, X., Li, J. and Singh, N. (2014) Recycling of spent lithium-ion battery: A critical review. Critical Reviews in Environmental Science and Technology, 44(10), 1129-1165. doi:10.1080/10643389.2013.763578
[8]. Yuan, C., Zhang, H. C. and Dornfeld, D. A. (2012). A three-dimensional framework for evaluating the sustainability of manufacturing processes. Journal of Manufacturing Science and Engineering, 134(2), 021013. doi:10.1115/1.4005674
[9]. Pillot, C. (2019). The rechargeable battery market and main trends 2018–2030. In 18th European Conference on Batteries, 1-14.
[10]. Chen, M., Ma, X., Chen, B. and Cheng, W. (2021). Circular economy and environmental sustainability: The role of multinational enterprises in the global value chain. Journal of Environmental Management, 287, 112307. doi:10.1016/j.jenvman.2021.112307
Cite this article
Liang,Y. (2024). Traditional and Innovative Battery Recycling Methods. Advances in Economics, Management and Political Sciences,123,42-48.
Data availability
The datasets used and/or analyzed during the current study will be available from the authors upon reasonable request.
Disclaimer/Publisher's Note
The statements, opinions and data contained in all publications are solely those of the individual author(s) and contributor(s) and not of EWA Publishing and/or the editor(s). EWA Publishing and/or the editor(s) disclaim responsibility for any injury to people or property resulting from any ideas, methods, instructions or products referred to in the content.
About volume
Volume title: Proceedings of ICEMGD 2024 Workshop: Policies to Enhance Sustainable Development through the Green Economy
© 2024 by the author(s). Licensee EWA Publishing, Oxford, UK. This article is an open access article distributed under the terms and
conditions of the Creative Commons Attribution (CC BY) license. Authors who
publish this series agree to the following terms:
1. Authors retain copyright and grant the series right of first publication with the work simultaneously licensed under a Creative Commons
Attribution License that allows others to share the work with an acknowledgment of the work's authorship and initial publication in this
series.
2. Authors are able to enter into separate, additional contractual arrangements for the non-exclusive distribution of the series's published
version of the work (e.g., post it to an institutional repository or publish it in a book), with an acknowledgment of its initial
publication in this series.
3. Authors are permitted and encouraged to post their work online (e.g., in institutional repositories or on their website) prior to and
during the submission process, as it can lead to productive exchanges, as well as earlier and greater citation of published work (See
Open access policy for details).