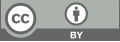
The Use of Metallic Elements in Bone Repair Scaffolds
- 1 Haier School,Qingdao,266001,China
* Author to whom correspondence should be addressed.
Abstract
Bone repair has been a challenging issue for a long time. Some recent studies focused on the incorporation of metallic elements into scafffolds for improving the performance for bone regeneration. This review explores the use of metallic elements in bone repair scaffolds, focusing on strontium (Sr), silicon (Si), magnesium (Mg) and Titanium (Ti). These elements enhance scaffold properties, promoting bone regeneration. Sr5(PO4)2SiO4 (SPS) bioceramic scaffolds, fabricated through 3D plotting and sol-gel methods, exhibit superior mechanical strength and induce osteogenesis and angiogenesis in vitro. Sr and Si ions within the SPS scaffolds upregulate genes related to cell proliferation, osteogenesis, and angiogenesis. Porous magnesium (Mg) scaffolds, with their biodegradability and ability to stimulate bone formation, offer an alternative to traditional metal implants. Mg scaffolds demonstrate good biocompatibility, physical properties, and osteoinductive potential. Porous titanium (Ti) scaffolds, manufactured through powder metallurgy, address the issue of stress shielding associated with conventional Ti implants. The interconnected pore network and controlled porosity of these scaffolds mimic natural bone, leading to improved biocompatibility and cell interaction.
Keywords
Bone repair scaffolds, strontium (Sr), silicon (Si), magnesium (Mg), titanium (Ti).
[1]. Amini, A. R., Laurencin, C. T., & Nukavarapu, S. P. (2012). Bone tissue engineering: recent advances and challenges. Critical Reviews in Biomedical Engineering, 40(5), 363-408.
[2]. Lichte, P., Pape, H. C., Pufe, T., Kobbe, P., & Fischer, H. (2011). Scaffolds for bone healing: concepts, materials and evidence. Injury, 42(6), 569-573.
[3]. N. Neves, D. Linhares, G. Costa, C.C. Ribeiro, M.A. Barbosa, In vivo and clinical application of strontium-enriched biomaterials for bone regeneration: a systematic review, Bone. Joint. Res. 6 (6) (2017) 366–375.
[4]. C. Wu, Z. Chen, D. Yi, J. Chang, Y. Xiao, Multidirectional effects of Sr-, Mg-, and Sicontaining bioceramic coatings with high bonding strength on inflammation, osteoclastogenesis and osteogenesis, ACS Appl. Mater. Interfaces. 6(6)(2014)4264–4276.
[5]. Zhu, H., Zhai, D., Lin, C., Zhang, Y., Huan, Z., Chang, J., & Wu, C. (2016). 3D plotting of highly uniform Sr5(PO4)2SiO4 bioceramic scaffolds for bone tissue engineering. Journal of Materials Chemistry B, 4(37), 6200-6212.
[6]. R. Jugdaohsingh, K.L. Tucker, N. Qiao, L.A. Cupples, D.P. Kiel, J.J. Powell, Dietary silicon intake is positively associated with bone mineral density in men and premenopausal women of the Framingham Offspring cohort, J. Bone. Miner. Res. 19 (2) (2004) 297–307.
[7]. D.M. Reffitt, N. Ogston, R. Jugdaohsingh, H.F.J. Cheung, B.A.J. Evans, R.P.H. Thompson, J.J. Powell, G.N. Hampson, Orthosilicic acid stimulates collagen type 1 synthesis and osteoblastic differentiation in human osteoblast-like cells in vitro, Bone.32 (2) (2003) 127–135.
[8]. Bohner, M. (2009). Silicon-substituted calcium phosphates–a critical view. Biomaterials, 30(32), 6403-6406.
[9]. Hing, K. A., Revell, P. A., Smith, N., & Buckland, T. (2006). Effect of silicon level on rate, quality and progression of bone healing within silicate-substituted porous hydroxyapatite scaffolds. Biomaterials, 27(29), 5014-5026.
[10]. Udduttula, A., Teng, B., Chandrashekar, B. N., Li, J., Yu, X. F., Liu, C., ... & Ren, P. G. (2020). Novel Sr5 (PO4) 2SiO4-graphene nanocomposites for applications in bone regeneration in vitro. Applied Surface Science, 507, 145176.
[11]. K. Maciejewska, Z. Drzazga, M. Kaszuba, Role of trace elements (Zn, Sr, Fe) in bone development: Energy dispersive X-ray fluorescence study of rat bone and tooth tissue, BioFactors. 40 (4) (2014) 425–435.
[12]. V. Mourino, J.P. Cattalini, A.R. Boccaccini, Metallic ions as therapeutic agents in tissue engineering scaffolds: an overview of their biological applications and strategies for new developments, J. Royal Soc. Interface. 9 (68) (2011) 401–419.
[13]. D.G. Yu, H.F. Ding, Y.Q. Mao, M. Liu, B. Yu, X. Zhao, X.Q. Wang, Y. Li, G.W. Liu, S.B. Nie, S. Liu, Strontium ranelate reduces cartilage degeneration and subchondral bone remodeling in rat osteoarthritis model, Acta. Pharmacol. Sin. 34 (3) (2013)393–402.
[14]. Huang, Y., Gan, J., & Seo, H. J. (2011). Luminescence investigation of Eu-activated Sr5 (PO4) 2SiO4 phosphor by combustion synthesis. Journal of the American Ceramic Society, 94(4), 1143-1148.
[15]. Y. Zheng, G. Dong, C. Deng, Effect of silicon content on the surface morphology of silicon-substituted hydroxyapatite bio-ceramics treated by a hydrothermal vapor method, Ceram. Int. 40 (2014) 14661–14667.
[16]. Udduttula, A., Li, J., Zhao, P. Y., Wang, G. C., Zhang, J. V., & Ren, P. G. (2019). Sol-gel derived nanosized Sr5 (PO4) 2SiO4 powder with enhanced in vitro osteogenesis and angiogenesis for bone regeneration applications. Ceramics International, 45(3), 3148-3158.
[17]. F. Witte, The history of biodegradable magnesium implants: a review, Acta Bio-mater. 6 (2010) 1680–1692.
[18]. C. Palacios, The role of nutrients in bone health, from A to Z, Crit. Rev. Food Sci. Nutr. 46 (2006) 621–628.
[19]. Y. Zhang, J. Xu, Y.C. Ruan, M.K. Yu, M. O’laughlin, H. Wise, D. Chen, L. Tian, D. Shi, J. Wang, S. Chen, J.Q. Feng, D.H. Chow, X. Xie, L. Zheng, L. Huang, S.Huang, K. Leung, N. Lu, L. Zhao, H. Li, D. Zhao, X. Guo, K. Chan, F. Witte, H.C. Chan, Y. Zheng, L. Qin, Implant-derived magnesium induces local neuronal production of CGRP to improve bone-fracture healing in rats, Nat. Med. 22 (2016) 1160–1169.
[20]. H. Hornberger, S. Virtanen, A. Boccaccini, Biomedical coatings on magnesium alloys–a review, Acta Biomater. 8 (2012) 2442–2455.
[21]. M. Razavi, M. Fathi, O. Savabi, D. Vashaee, L. Tayebi, Biodegradation, bioactivity and in vivo biocompatibility analysis of plasma electrolytic oxidized (PEO) biodegradable Mg implants, Phys. Sci. Int. J. 4 (2014) 708–722.
[22]. Gray JE, Luan B. Protective coatings on magnesium and its alloys - a critical review. J Alloys Compd 2002; 336: 88-113, doi: 10.1016/S0925-8388(01)01899-0.
[23]. Liu, Y. J., Yang, Z. Y., Tan, L. L., Li, H., & Zhang, Y. Z. (2014). An animal experimental study of porous magnesium scaffold degradation and osteogenesis. Brazilian Journal of Medical and Biological Research, 47(8), 715-720.
[24]. Lin, T., Wang, X., Jin, L., Li, W., Zhang, Y., Wang, A., ... & Shao, H. (2021). Manufacturing of porous magnesium scaffolds for bone tissue engineering by 3D gel-printing. Materials & Design, 209, 109948.
[25]. Yu, G., Li, Z., Li, S., Zhang, Q., Hua, Y., Liu, H., ... & Wang, X. (2020). The select of internal architecture for porous Ti alloy scaffold: A compromise between mechanical properties and permeability. Materials & Design, 192, 108754.
[26]. Dabrowski, B., Swieszkowski, W., Godlinski, D., & Kurzydlowski, K. J. (2010). Highly porous titanium scaffolds for orthopaedic applications. Journal of Biomedical Materials Research Part B: Applied Biomaterials, 95(1), 53-61.
[27]. Loh Q L and Choong C 2013 Three-dimensional scaffolds for tissue engineering applications: role of porosity and pore size. Tissue Eng. Part B. Rev. 19 485–502
[28]. Chen, Y., Frith, J. E., Dehghan-Manshadi, A., Attar, H., Kent, D., Soro, N. D. M., ... & Dargusch, M. S. (2017). Mechanical properties and biocompatibility of porous titanium scaffolds for bone tissue engineering. Journal of the mechanical behavior of biomedical materials, 75, 169-174.
[29]. Elias, C. N., Lima, J. H. C., Valiev, R., & Meyers, M. A. (2008). Biomedical applications of titanium and its alloys. Jom, 60, 46-49.
Cite this article
Sun,Z. (2024). The Use of Metallic Elements in Bone Repair Scaffolds. Theoretical and Natural Science,63,38-44.
Data availability
The datasets used and/or analyzed during the current study will be available from the authors upon reasonable request.
Disclaimer/Publisher's Note
The statements, opinions and data contained in all publications are solely those of the individual author(s) and contributor(s) and not of EWA Publishing and/or the editor(s). EWA Publishing and/or the editor(s) disclaim responsibility for any injury to people or property resulting from any ideas, methods, instructions or products referred to in the content.
About volume
Volume title: Proceedings of the 4th International Conference on Biological Engineering and Medical Science
© 2024 by the author(s). Licensee EWA Publishing, Oxford, UK. This article is an open access article distributed under the terms and
conditions of the Creative Commons Attribution (CC BY) license. Authors who
publish this series agree to the following terms:
1. Authors retain copyright and grant the series right of first publication with the work simultaneously licensed under a Creative Commons
Attribution License that allows others to share the work with an acknowledgment of the work's authorship and initial publication in this
series.
2. Authors are able to enter into separate, additional contractual arrangements for the non-exclusive distribution of the series's published
version of the work (e.g., post it to an institutional repository or publish it in a book), with an acknowledgment of its initial
publication in this series.
3. Authors are permitted and encouraged to post their work online (e.g., in institutional repositories or on their website) prior to and
during the submission process, as it can lead to productive exchanges, as well as earlier and greater citation of published work (See
Open access policy for details).