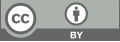
Immunomodulatory Effects of Breast Cancer Therapies: Unraveling the Crosstalk Between Therapeutics and the Tumor Immune Microenvironment
- 1 Institute of Pharmacy, Macau University of Science and Technology, Avendia Wai Long, Taipa, Macau
* Author to whom correspondence should be addressed.
Abstract
Breast cancer is a heterogeneous malignancy that consists of multiple molecular subtypes, each requiring therapeutic approaches distinctively. While traditional treatments, including endocrine therapy, and anti-HER2 therapy, primarily targeting on cancer cell proliferation and survival, emerging evidence underscores their profound immunomodulatory effects within the tumor microenvironment. The intricate interplay between breast cancer treatments and immune cells shapes therapeutic efficacy and patient outcomes. This review aiming to analysis the immunomodulatory functions of various therapies, including selective estrogen receptor modulators (SERMs), aromatase inhibitors (AIs), and CDK4/6 inhibitors; monoclonal antibodies, tyrosine kinase inhibitors (TKIs), and antibody-drug conjugates (ADCs); and the role of PARP inhibitors, PI3K inhibitors, and mTOR inhibitors in modulating immune responses. We discuss how these treatments impact key immune populations, including tumor-infiltrating lymphocytes, myeloid-derived suppressor cells (MDSCs), and natural killer (NK) cells, as well as their influence on immune checkpoints and cytokine signaling pathways. Understanding the dual role of breast cancer therapies in both suppressing tumor growth and modulating the immune landscape offers new opportunities for strategies integrating targeted therapy and immunotherapy. By elucidating these interactions, we aim to provide insights into optimizing treatment strategies to enhance antitumor immunity and improve patient prognosis.
Keywords
Breast cancer, Immune tumor environment, Therapeutics Immunomodulation
[1]. Siegel R L, Miller K D, Jemal A. Cancer statistics, 2019[J]. CA: a cancer journal for clinicians, 2019, 69(1): 7-34.
[2]. Hankinson S E, Colditz G A, Willett W C. Towards an integrated model for breast cancer etiology: the lifelong interplay of genes, lifestyle, and hormones[J]. Breast Cancer Research, 2004, 6: 1-6.
[3]. Danaei G, Vander Hoorn S, Lopez A D, et al. Causes of cancer in the world: comparative risk assessment of nine behavioural and environmental risk factors[J]. The lancet, 2005, 366(9499): 1784-1793.
[4]. Perou C M, Sørlie T, Eisen M B, et al. Molecular portraits of human breast tumours[J]. nature, 2000, 406(6797): 747-752.
[5]. Breast cancer Loibl, Sibylle et al. The Lancet, Volume 397, Issue 10286, 1750 - 1769
[6]. Prat A, Perou C M. Mammary development meets cancer genomics[J]. Nature medicine, 2009, 15(8): 842-844.
[7]. Burstein H J, Temin S, Anderson H, et al. Adjuvant endocrine therapy for women with hormone receptor–positive breast cancer: American Society of Clinical Oncology clinical practice guideline focused update[J]. Journal of clinical oncology, 2014, 32(21): 2255-2269.
[8]. Hicks D G, Kulkarni S. HER2+ breast cancer: review of biologic relevance and optimal use of diagnostic tools[J]. American journal of clinical pathology, 2008, 129(2): 263-273.
[9]. Yarden Y. Biology of HER2 and its importance in breast cancer[J]. Oncology, 2001, 61(Suppl. 2): 1-13.
[10]. Gianni L, Pienkowski T, Im Y H, et al. 5-year analysis of neoadjuvant pertuzumab and trastuzumab in patients with locally advanced, inflammatory, or early-stage HER2-positive breast cancer (NeoSphere): a multicentre, open-label, phase 2 randomised trial[J]. The lancet oncology, 2016, 17(6): 791-800.
[11]. Von Minckwitz G, Procter M, De Azambuja E, et al. Adjuvant pertuzumab and trastuzumab in early HER2-positive breast cancer[J]. New England Journal of Medicine, 2017, 377(2): 122-131.
[12]. Corona S P, Ravelli A, Cretella D, et al. CDK4/6 inhibitors in HER2-positive breast cancer[J]. Critical reviews in oncology/hematology, 2017, 112: 208-214.
[13]. Xie B, Zhu L, Ma C, et al. A network meta-analysis on the efficacy of HER2-targeted agents in combination with taxane-containing regimens for treatment of HER2-positive metastatic breast cancer[J]. Breast Cancer, 2020, 27: 186-196.
[14]. Lehmann B D, Bauer J A, Chen X, et al. Identification of human triple-negative breast cancer subtypes and preclinical models for selection of targeted therapies[J]. The Journal of clinical investigation, 2011, 121(7): 2750-2767.
[15]. Wang, DY., Jiang, Z., Ben-David, Y. et al. Molecular stratification within triple-negative breast cancer subtypes. Sci Rep 9, 19107 (2019).
[16]. He J, McLaughlin R P, van der Noord V, et al. Multi-targeted kinase inhibition alleviates mTOR inhibitor resistance in triple-negative breast cancer[J]. Breast Cancer Research and Treatment, 2019, 178: 263-274.
[17]. Vtorushin S, Dulesova A, Krakhmal N. Luminal androgen receptor (LAR) subtype of triple-negative breast cancer: molecular, morphological, and clinical features[J]. Journal of Zhejiang University-SCIENCE B, 2022, 23(8): 617-624.
[18]. Lee J M, Ledermann J A, Kohn E C. PARP Inhibitors for BRCA1/2 mutation-associated and BRCA-like malignancies[J]. Annals of oncology, 2014, 25(1): 32-40.
[19]. Timms K M, Abkevich V, Hughes E, et al. Association of BRCA1/2 defects with genomic scores predictive of DNA damage repair deficiency among breast cancer subtypes[J]. Breast Cancer Research, 2014, 16: 1-9.
[20]. Wen W X, Leong C O. Association of BRCA1-and BRCA2-deficiency with mutation burden, expression of PD-L1/PD-1, immune infiltrates, and T cell-inflamed signature in breast cancer[J]. PLoS One, 2019, 14(4): e0215381.
[21]. Kythreotou A, Siddique A, Mauri F A, et al. PD-L1[J]. Journal of clinical pathology, 2018, 71(3): 189-194.
[22]. Han Y, Liu D, Li L. PD-1/PD-L1 pathway: current researches in cancer[J]. American journal of cancer research, 2020, 10(3): 727.
[23]. Li C J, Lin L T, Hou M F, et al. PD‑L1/PD‑1 blockade in breast cancer: The immunotherapy era[J]. Oncology Reports, 2021, 45(1): 5-12.
[24]. Schütz F, Stefanovic S, Mayer L, et al. PD-1/PD-L1 pathway in breast cancer[J]. Oncology research and treatment, 2017, 40(5): 294-297.
[25]. Schmieder A, Michel J, Schönhaar K, et al. Differentiation and gene expression profile of tumor-associated macrophages[C]//Seminars in cancer biology. Academic Press, 2012, 22(4): 289-297.
[26]. Matusz-Fisher A, Tan A R. Combination of HER2-targeted agents with immune checkpoint inhibitors in the treatment of HER2-positive breast cancer[J]. Expert Opinion on Biological Therapy, 2022, 22(3): 385-395.
[27]. Vesely M D, Kershaw M H, Schreiber R D, et al. Natural innate and adaptive immunity to cancer[J]. Annual review of immunology, 2011, 29(1): 235-271.
[28]. Place A E, Jin Huh S, Polyak K. The microenvironment in breast cancer progression: biology and implications for treatment[J]. Breast cancer research, 2011, 13: 1-11.
[29]. Vesely M D, Kershaw M H, Schreiber R D, et al. Natural innate and adaptive immunity to cancer[J]. Annual review of immunology, 2011, 29(1): 235-271.
[30]. Kovats S. Estrogen receptors regulate innate immune cells and signaling pathways[J]. Cellular immunology, 2015, 294(2): 63-69.
[31]. Chakraborty B, Byemerwa J, Krebs T, et al. Estrogen receptor signaling in the immune system[J]. Endocrine reviews, 2023, 44(1): 117-141.
[32]. Silva C M, Isgaard J, Thorner M O. Cytokines in endocrine function[J]. Advances in protein chemistry, 1998, 52: 199-221.
[33]. Riggs B L, Hartmann L C. Selective estrogen-receptor modulators—mechanisms of action and application to clinical practice[J]. New England Journal of Medicine, 2003, 348(7): 618-629.
[34]. Chan K K L, Wei N, Liu S S, et al. Estrogen receptor subtypes in ovarian cancer: a clinical correlation[J]. Obstetrics & Gynecology, 2008, 111(1): 144-151.
[35]. Smith D, Stewart C J R, Clarke E M, et al. ER and PR expression and survival after endometrial cancer[J]. Gynecologic oncology, 2018, 148(2): 258-266.
[36]. Chang M. Tamoxifen resistance in breast cancer[J]. Biomolecules & therapeutics, 2012, 20(3): 256.
[37]. Behjati S, Frank M H. The effects of tamoxifen on immunity[J]. Current medicinal chemistry, 2009, 16(24): 3076-3080.
[38]. Bebo Jr B F, Dehghani B, Foster S, et al. Treatment with selective estrogen receptor modulators regulates myelin specific T‐cells and suppresses experimental autoimmune encephalomyelitis[J]. Glia, 2009, 57(7): 777-790.
[39]. Robinson E, Rubin D, Mekori T, et al. In vivo modulation of natural killer cell activity by tamoxifen in patients with bilateral primary breast cancer[J]. Cancer Immunology, Immunotherapy, 1993, 37: 209-212.
[40]. Schild-Hay L J, Leil T A, Divi R L, et al. Tamoxifen Induces Expression of Immune Response–Related Genes in Cultured Normal Human Mammary Epithelial Cells[J]. Cancer research, 2009, 69(3): 1150-1155.
[41]. Huang H, Zhou J, Chen H, et al. The immunomodulatory effects of endocrine therapy in breast cancer[J]. Journal of Experimental & Clinical Cancer Research, 2021, 40: 1-16.
[42]. Smith I E, Dowsett M. Aromatase inhibitors in breast cancer[J]. New England Journal of Medicine, 2003, 348(24): 2431-2442.
[43]. Chumsri S, Howes T, Bao T, et al. Aromatase, aromatase inhibitors, and breast cancer[J]. The Journal of steroid biochemistry and molecular biology, 2011, 125(1-2): 13-22.
[44]. Scott L J, Wiseman L R. Exemestane[J]. Drugs, 1999, 58: 675-680.
[45]. Pardhi M, Mehsram P, Bamgude P, et al. Synthesis of Chalcone Derivatives and its Biological Evaluations: An Overview[J]. 2025.
[46]. Kelly C M, Buzdar A U. Anastrozole[J]. Expert opinion on drug safety, 2010, 9(6): 995-1003.
[47]. Bhatnagar A S. The discovery and mechanism of action of letrozole[J]. Breast cancer research and treatment, 2007, 105(Suppl 1): 7-17.
[48]. Sabale P M, Sabale V P, Potey L C. Aromatase and aromatase inhibitors in breast cancer treatment[J]. J. Curr. Pharma Res., 2018, 9: 2636-2655.
[49]. Edris A, Abdelrahman M, Osman W, et al. Design of novel letrozole analogues targeting aromatase for breast cancer: molecular docking, molecular dynamics, and theoretical studies on gold nanoparticles[J]. Metabolites, 2023, 13(5): 583.
[50]. Zhao R, Chen C, Zhen L. Effects of zoledronic acid and exemestane combination therapy on immune function, sex hormones, bone markers, and clinical efficacy in hormone receptor-positive breast cancer patients[J]. European Journal of Gynaecological Oncology, 2024, 45(2).
[51]. Ma C X, Reinert T, Chmielewska I, et al. Mechanisms of aromatase inhibitor resistance[J]. Nature Reviews Cancer, 2015, 15(5): 261-275.
[52]. Generali D, Bates G, Berruti A, et al. Immunomodulation of FOXP3+ regulatory T cells by the aromatase inhibitor letrozole in breast cancer patients[J]. Clinical Cancer Research, 2009, 15(3): 1046-1051.
[53]. Bui K T, Willson M L, Goel S, et al. Ovarian suppression for adjuvant treatment of hormone receptor‐positive early breast cancer[J]. Cochrane Database of Systematic Reviews, 1996, 2020(3).
[54]. Francis P A, Regan M M, Fleming G F, et al. Adjuvant ovarian suppression in premenopausal breast cancer[J]. New England Journal of Medicine, 2015, 372(5): 436-446.
[55]. Millar R P. GnRHs and GnRH receptors[J]. Animal reproduction science, 2005, 88(1-2): 5-28.
[56]. Montgomery R A, Dallman M J. Analysis of cytokine gene expression during fetal thymic ontogeny using the polymerase chain reaction[J]. Journal of immunology (Baltimore, Md.: 1950), 1991, 147(2): 554-560.
[57]. Zakharova L, Sharova V, Izvolskaia M. Mechanisms of reciprocal regulation of gonadotropin-releasing hormone (GnRH)-producing and immune systems: the role of GnRH, cytokines and their receptors in early ontogenesis in normal and pathological conditions[J]. International journal of molecular sciences, 2020, 22(1): 114. receptors in early ontogenesis in normal and pathological conditions[J]. International Journal of Molecular Sciences, 2020, 22(1): 114.
[58]. Tanriverdi F, Gonzalez-Martinez D, Silveira L F G, et al. Expression of gonadotropin-releasing hormone type-I (GnRH-I) and type-II (GnRH-II) in human peripheral blood mononuclear cells (PMBCs) and regulation of B-lymphoblastoid cell proliferation by GnRH-I and GnRH-II[J]. Experimental and clinical endocrinology & diabetes, 2004, 112(10): 587-594. [59] Rao L V, Cleveland R P, Kimmel R J, et al. Hematopoietic Stem Cell Antigen‐1 (Sca‐1) Expression in Different Lymphoid Tissues of Female Mice Treated With GnRH Agonist[J]. American Journal of Reproductive Immunology, 1995, 34(4): 257-266.
[59]. Sherr C J, Beach D, Shapiro G I. Targeting CDK4 and CDK6: from discovery to therapy[J]. Cancer discovery, 2016, 6(4): 353-367.
[60]. Deng J, Wang E S, Jenkins R W, et al. CDK4/6 inhibition augments antitumor immunity by enhancing T-cell activation[J]. Cancer discovery, 2018, 8(2): 216-233.
[61]. Veinotte L, Gebremeskel S, Johnston B. CXCL16-positive dendritic cells enhance invariant natural killer T cell-dependent IFNγ production and tumor control[J]. Oncoimmunology, 2016, 5(6): e1160979.
[62]. Peng D, Kryczek I, Nagarsheth N, et al. Epigenetic silencing of TH1-type chemokines shapes tumour immunity and immunotherapy[J]. Nature, 2015, 527(7577): 249-253.
[63]. Patsoukis N, Wang Q, Strauss L, et al. Revisiting the PD-1 pathway[J]. Science advances, 2020, 6(38): eabd2712.
[64]. Schütz F, Stefanovic S, Mayer L, et al. PD-1/PD-L1 pathway in breast cancer[J]. Oncology research and treatment, 2017, 40(5): 294-297.
[65]. Ross J S, Slodkowska E A, Symmans W F, et al. The HER-2 receptor and breast cancer: ten years of targeted anti–HER-2 therapy and personalized medicine[J]. The oncologist, 2009, 14(4): 320-368.
[66]. Lopez-Albaitero A, Xu H, Guo H, et al. Overcoming resistance to HER2-targeted therapy with a novel HER2/CD3 bispecific antibody[J]. Oncoimmunology, 2017, 6(3): e1267891.
[67]. Boekhout A H, Beijnen J H, Schellens J H M. Trastuzumab[J]. The oncologist, 2011, 16(6): 800-810.
[68]. Capelan M, Pugliano L, De Azambuja E, et al. Pertuzumab: new hope for patients with HER2-positive breast cancer[J]. Annals of oncology, 2013, 24(2): 273-282.
[69]. Park J W, Kirpotin D B, Hong K, et al. Tumor targeting using anti-her2 immunoliposomes[J]. Journal of controlled release, 2001, 74(1-3): 95-113.
[70]. Musolino A, Gradishar W J, Rugo H S, et al. Role of Fcγ receptors in HER2-targeted breast cancer therapy[J]. Journal for immunotherapy of cancer, 2022, 10(1): e003171.
[71]. Park S G, Jiang Z, Mortenson E D, et al. The therapeutic effect of anti-HER2/neu antibody depends on both innate and adaptive immunity[J]. Cancer cell, 2010, 18(2): 160-170.
[72]. Jaime-Ramirez A C, Mundy-Bosse B L, Kondadasula S V, et al. IL-12 enhances the antitumor actions of trastuzumab via NK cell IFN-γ production[J]. The Journal of Immunology, 2011, 186(6): 3401-3409.
[73]. Vignali D A A, Kuchroo V K. IL-12 family cytokines: immunological playmakers[J]. Nature immunology, 2012, 13(8): 722-728.
[74]. Deguine J, Barton G M. MyD88: a central player in innate immune signaling[J]. F1000prime reports, 2014, 6: 97.
[75]. Nuti M, Bellati F, Visconti V, et al. Immune effects of trastuzumab[J]. Journal of Cancer, 2011, 2: 317.
[76]. Benavides L C, Sears A K, Gates J D, et al. Comparison of different HER2/neu vaccines in adjuvant breast cancer trials: implications for dosing of peptide vaccines[J]. Expert review of vaccines, 2011, 10(2): 201-210.
[77]. Schlam I, Swain S M. HER2-positive breast cancer and tyrosine kinase inhibitors: the time is now[J]. NPJ breast cancer, 2021, 7(1): 56.
[78]. Xuhong J C, Qi X W, Zhang Y, et al. Mechanism, safety and efficacy of three tyrosine kinase inhibitors lapatinib, neratinib and pyrotinib in HER2-positive breast cancer[J]. American journal of cancer research, 2019, 9(10): 2103.
[79]. Gotink K J, Verheul H M W. Anti-angiogenic tyrosine kinase inhibitors: what is their mechanism of action?[J]. Angiogenesis, 2010, 13: 1-14.
[80]. Garrett J T, Arteaga C L. Resistance to HER2-directed antibodies and tyrosine kinase inhibitors: mechanisms and clinical implications[J]. Cancer biology & therapy, 2011, 11(9): 793-800.
[81]. Hannesdóttir L, Tymoszuk P, Parajuli N, et al. Lapatinib and doxorubicin enhance the S tat1‐dependent antitumor immune response[J]. European journal of immunology, 2013, 43(10): 2718-2729.
[82]. Fukai S, Nakajima S, Saito M, et al. Down-regulation of stimulator of interferon genes (STING) expression and CD8+ T-cell infiltration depending on HER2 heterogeneity in HER2-positive gastric cancer[J]. Gastric Cancer, 2023, 26(6): 878-890.
[83]. Hopfner K P, Hornung V. Molecular mechanisms and cellular functions of cGAS–STING signalling[J]. Nature reviews Molecular cell biology, 2020, 21(9): 501-521.
[84]. Showalter L E, Oechsle C, Ghimirey N, et al. Th1 cytokines sensitize HER-expressing breast cancer cells to lapatinib[J]. PLoS One, 2019, 14(1): e0210209.
[85]. Hannesdóttir L, Tymoszuk P, Parajuli N, et al. Lapatinib and doxorubicin enhance the S tat1‐dependent antitumor immune response[J]. European journal of immunology, 2013, 43(10): 2718-2729.
[86]. Rinnerthaler G, Gampenrieder S P, Greil R. HER2 directed antibody-drug-conjugates beyond T-DM1 in breast cancer[J]. International journal of molecular sciences, 2019, 20(5): 1115.
[87]. Krop I, Winer E P. Trastuzumab emtansine: a novel antibody–drug conjugate for HER2-positive breast cancer[J]. Clinical Cancer Research, 2014, 20(1): 15-20.
[88]. Hurvitz S, Kim S B, Chung W P, et al. Abstract GS3-01: Trastuzumab deruxtecan (T-DXd; DS-8201a) vs. trastuzumab emtansine (T-DM1) in patients (pts) with HER2+ metastatic breast cancer (mBC): subgroup analyses from the randomized phase 3 study DESTINY-Breast03[J]. Cancer Research, 2022, 82(4_Supplement): GS3-01-GS3-01.
[89]. Uppal H, Doudement E, Mahapatra K, et al. Potential mechanisms for thrombocytopenia development with trastuzumab emtansine (T-DM1)[J]. Clinical Cancer Research, 2015, 21(1): 123-133.
[90]. Liu F, Mao K, Chen H, et al. Enhancing the Safety and Efficacy of Trastuzumab Emtansine (T‐DM1) Through Nano‐Delivery System in Breast Cancer Therapy[J]. Small, 2024, 20(50): 2400977.
[91]. Hunter F W, Barker H R, Lipert B, et al. Mechanisms of resistance to trastuzumab emtansine (T-DM1) in HER2-positive breast cancer[J]. British journal of cancer, 2020, 122(5): 603-612.
[92]. Kim R. Cancer immunoediting: from immune surveillance to immune escape[J]. Cancer Immunotherapy, 2007: 9-27.
[93]. André F, Ciruelos E, Rubovszky G, et al. Alpelisib for PIK3CA-mutated, hormone receptor–positive advanced breast cancer[J]. New England Journal of Medicine, 2019, 380(20): 1929-1940.
[94]. Robson M, Im S A, Senkus E, et al. Olaparib for metastatic breast cancer in patients with a germline BRCA mutation[J]. New England Journal of Medicine, 2017, 377(6): 523-533.
[95]. Piccart M, Hortobagyi G N, Campone M, et al. Everolimus plus exemestane for hormone-receptor-positive, human epidermal growth factor receptor-2-negative advanced breast cancer: overall survival results from BOLERO-2[J]. Annals of oncology, 2014, 25(12): 2357-2362.
[96]. Yan C, Yang J, Saleh N, et al. Inhibition of the PI3K/mTOR pathway in breast cancer to enhance response to immune checkpoint inhibitors in breast cancer[J]. International journal of molecular sciences, 2021, 22(10): 5207.
[97]. André F, Ciruelos E, Rubovszky G, et al. Alpelisib for PIK3CA-mutated, hormone receptor–positive advanced breast cancer[J]. New England Journal of Medicine, 2019, 380(20): 1929-1940.
[98]. Pompura S L, Dominguez-Villar M. The PI3K/AKT signaling pathway in regulatory T-cell development, stability, and function[J]. Journal of leukocyte biology, 2018, 103(6): 1065-1076.
[99]. Armaghani A J, Han H S. Alpelisib in the treatment of breast cancer: a short review on the emerging clinical data[J]. Breast Cancer: Targets and Therapy, 2020: 251-258.
[100]. Li X, Chen G, Wang F, et al. Oncogenic PIK3CA recruits myeloid‐derived suppressor cells to shape the immunosuppressive tumour microenvironment in luminal breast cancer through the 5‐lipoxygenase‐dependent arachidonic acid pathway[J]. Clinical and Translational Medicine, 2023, 13(11): e1483.
[101]. Stark A K, Davenport E, Patton D T, et al. Loss of phosphatidylinositol 3-kinase activity in regulatory T cells leads to neuronal inflammation[J]. The Journal of Immunology, 2020, 205(1): 78-89.
[102]. Sun P, Zhang X, Wang R J, et al. PI3Kα inhibitor CYH33 triggers antitumor immunity in murine breast cancer by activating CD8+ T cells and promoting fatty acid metabolism[J]. Journal for ImmunoTherapy of Cancer, 2021, 9(8): e003093.
[103]. Drullinsky P R, Hurvitz S A. Mechanistic basis for PI3K inhibitor antitumor activity and adverse reactions in advanced breast cancer[J]. Breast cancer research and treatment, 2020, 181: 233-248.
[104]. Griguolo G, Dieci M V, Guarneri V, et al. Olaparib for the treatment of breast cancer[J]. Expert review of anticancer therapy, 2018, 18(6): 519-530.
[105]. Staniszewska A D, Armenia J, King M, et al. PARP inhibition is a modulator of anti-tumor immune response in BRCA-deficient tumors[J]. Oncoimmunology, 2022, 11(1): 2083755.
[106]. Pantelidou C, Sonzogni O, De Oliveria Taveira M, et al. PARP inhibitor efficacy depends on CD8+ T-cell recruitment via intratumoral STING pathway activation in BRCA-deficient models of triple-negative breast cancer[J]. Cancer discovery, 2019, 9(6): 722-737.
[107]. Pantelidou C, Sonzogni O, De Oliveria Taveira M, et al. PARP inhibitor efficacy depends on CD8+ T-cell recruitment via intratumoral STING pathway activation in BRCA-deficient models of triple-negative breast cancer[J]. Cancer discovery, 2019, 9(6): 722-737.
[108]. Lampert E J, Zimmer A, Padget M, et al. Combination of PARP inhibitor olaparib, and PD-L1 inhibitor durvalumab, in recurrent ovarian cancer: a proof-of-concept phase II study[J]. Clinical Cancer Research, 2020, 26(16): 4268-4279.
[109]. Jiao S, Xia W, Yamaguchi H, et al. PARP inhibitor upregulates PD-L1 expression and enhances cancer-associated immunosuppression[J]. Clinical Cancer Research, 2017, 23(14): 3711-3720.
[110]. Powell J D, Pollizzi K N, Heikamp E B, et al. Regulation of immune responses by mTOR[J]. Annual review of immunology, 2012, 30(1): 39-68.
[111]. Weichhart T, Hengstschläger M, Linke M. Regulation of innate immune cell function by mTOR[J]. Nature Reviews Immunology, 2015, 15(10): 599-614.
[112]. Baselga J, Campone M, Piccart M, et al. Everolimus in postmenopausal hormone-receptor–positive advanced breast cancer[J]. New England Journal of Medicine, 2012, 366(6): 520-529.
[113]. Hare S H, Harvey A J. mTOR function and therapeutic targeting in breast cancer[J]. American journal of cancer research, 2017, 7(3): 383.
[114]. Witzig T E, Reeder C, Han J J, et al. The mTORC1 inhibitor everolimus has antitumor activity in vitro and produces tumor responses in patients with relapsed T-cell lymphoma[J]. Blood, The Journal of the American Society of Hematology, 2015, 126(3): 328-335.
Cite this article
Xu,R. (2025). Immunomodulatory Effects of Breast Cancer Therapies: Unraveling the Crosstalk Between Therapeutics and the Tumor Immune Microenvironment. Theoretical and Natural Science,99,51-63.
Data availability
The datasets used and/or analyzed during the current study will be available from the authors upon reasonable request.
Disclaimer/Publisher's Note
The statements, opinions and data contained in all publications are solely those of the individual author(s) and contributor(s) and not of EWA Publishing and/or the editor(s). EWA Publishing and/or the editor(s) disclaim responsibility for any injury to people or property resulting from any ideas, methods, instructions or products referred to in the content.
About volume
Volume title: Proceedings of the 5th International Conference on Biological Engineering and Medical Science
© 2024 by the author(s). Licensee EWA Publishing, Oxford, UK. This article is an open access article distributed under the terms and
conditions of the Creative Commons Attribution (CC BY) license. Authors who
publish this series agree to the following terms:
1. Authors retain copyright and grant the series right of first publication with the work simultaneously licensed under a Creative Commons
Attribution License that allows others to share the work with an acknowledgment of the work's authorship and initial publication in this
series.
2. Authors are able to enter into separate, additional contractual arrangements for the non-exclusive distribution of the series's published
version of the work (e.g., post it to an institutional repository or publish it in a book), with an acknowledgment of its initial
publication in this series.
3. Authors are permitted and encouraged to post their work online (e.g., in institutional repositories or on their website) prior to and
during the submission process, as it can lead to productive exchanges, as well as earlier and greater citation of published work (See
Open access policy for details).