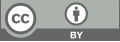
Interfacial Engineering in Solid-State Lithium Metal Batteries: Degradation Mechanisms and Dynamic Regulation Strategies
- 1 School of Chemistry and Molecular Engineering, East China University of Science and Technology, Shanghai 200237, China
* Author to whom correspondence should be addressed.
Abstract
Solid-state lithium metal batteries (SLMBs), recognized as next-generation energy storage systems for their high energy density and intrinsic safety, face commercialization challenges stemming from interfacial instability, lithium dendrite proliferation, and rapid capacity degradation in anode-free configurations. This review analyzes the underlying mechanisms of performance deterioration in SLMBs: (1) the highly reactive lithium metal engages in chemical or electrochemical side reactions with the solid-state electrolyte (SE), leading to a continuous increase in interfacial impedance; (2) Volume fluctuations during lithium deposition/stripping trigger mechanical contact failure; (3) Irreversible lithium consumption in anode-free systems creates a self-amplifying degradation cycle. In order to solve these issues, we focuses on three major modification strategies: (1) Three-dimensional porous current collectors for homogeneous lithium-ion flux regulation and dendrite suppression; (2) Constructing a gradient artificial interfacial layer to inhibit electron leakage and promote ion transport; (3) Developing dynamic self-adaptive interfacial systems that utilize in situ alloying reactions to achieve interface self-healing. In addition, the core contradictions in anode-free systems are furtherly analyzed. Furthermore, we emphasize the critical role of synergistic optimization between current collectors and interfacial layers in enhancing lithium deposition reversibility. By elucidating the fundamental principles and interdependencies of existing modification approaches, this review provides theoretical guidance and innovative design principles for developing high-performance SLMBs with extended cycle life and commercial viability.
Keywords
solid-state lithium metal batteries, anode-free, lithium dendrites, interfacial engineering, three-dimensional current collector
[1]. Lu, Y., Chen, H., Wang, L., Yu, Z., Huang, Y., Yu, X., Wang, Y. and Roskilly, A.P. (2021) Energy Storage Driving towards a Clean Energy Future. Energy Reports, 7, 8128-8130.
[2]. Schmuch, R., Wagner, R., Hörpel, G., Placke, T. and Winter, M. (2018) Performance and Cost of Materials for Lithium-Based Rechargeable Automotive Batteries. Nature Energy, 3, 267-278.
[3]. XU J, CAI X, CAI S, et al. High‐Energy Lithium‐Ion Batteries: Recent Progress and a Promising Future in Applications[J/OL]. ENERGY & ENVIRONMENTAL MATERIALS, 2023, 6(5): e12450. DOI:10.1002/eem2.12450.
[4]. Lin L, Qin K, Li M, et al. Spinel-related Li2Ni0. 5Mn1. 5O4 cathode for 5-V anode-free lithium metal batteries[J]. Energy Storage Materials, 2022, 45: 821-827.
[5]. Albertus P, Babinec S, Litzelman S, et al. Status and challenges in enabling the lithium metal electrode for high-energy and low-cost rechargeable batteries[J]. Nature Energy, 2018, 3(1): 16-21.
[6]. Yang C , Fu K , Zhang Y ,et al.Protected Lithium‐Metal Anodes in Batteries: From Liquid to Solid[J].Advanced Materials, 2017, 29(36):1701169.DOI:10.1002/adma.201701169.
[7]. Yang C, Xie H, Ping W, et al. An electron/ion dual‐conductive alloy framework for high‐rate and high‐capacity solid‐state lithium‐metal batteries[J]. Advanced Materials, 2019, 31(3): 1804815.
[8]. Huang W Z, Zhao C Z, Wu P, et al. Anode‐free solid‐state lithium batteries: a review[J]. Advanced Energy Materials, 2022, 12(26): 2201044.
[9]. Yu W, Lin K Y, Boyle D T, et al. Electrochemical formation of bis (fluorosulfonyl) imide-derived solid-electrolyte interphase at Li-metal potential[J]. Nature Chemistry, 2024: 1-10.
[10]. Ding J F, Xu R, Yan C, et al. A review on the failure and regulation of solid electrolyte interphase in lithium batteries[J]. Journal of Energy Chemistry, 2021, 59: 306-319.
[11]. Kim K J, Balaish M, Wadaguchi M, et al. Solid‐state Li–metal batteries: challenges and horizons of oxide and sulfide solid electrolytes and their interfaces[J]. Advanced Energy Materials, 2021, 11(1): 2002689.
[12]. Zhao D, Wang J, Wang P, et al. Regulating the composition distribution of layered SEI film on Li-ion battery anode by LiDFBOP[J]. Electrochimica Acta, 2020, 337: 135745.
[13]. Brooks D J, Merinov B V, Goddard III W A, et al. Atomistic description of ionic diffusion in PEO–LiTFSI: Effect of temperature, molecular weight, and ionic concentration[J]. Macromolecules, 2018, 51(21): 8987-8995.
[14]. Wang Q, Liu B, Shen Y, et al. Confronting the challenges in lithium anodes for lithium metal batteries[J]. Advanced Science, 2021, 8(17): 2101111.
[15]. Koerver R, Zhang W, De Biasi L, et al. Chemo-mechanical expansion of lithium electrode materials–on the route to mechanically optimized all-solid-state batteries[J]. Energy & Environmental Science, 2018, 11(8): 2142-2158.
[16]. Esmizadeh S, Cabras L, Serpelloni M, et al. A review on modeling of nucleation and growth of Li dendrites in solid electrolytes[J]. Journal of Energy Storage, 2024, 97: 112897.
[17]. Wu B, Wang S, Lochala J, et al. The role of the solid electrolyte interphase layer in preventing Li dendrite growth in solid-state batteries[J]. Energy & Environmental Science, 2018, 11(7): 1803-1810.
[18]. Krauskopf T, Richter F H, Zeier W G, et al. Physicochemical concepts of the lithium metal anode in solid-state batteries[J]. Chemical reviews, 2020, 120(15): 7745-7794.
[19]. Jagger B, Pasta M. Solid electrolyte interphases in lithium metal batteries[J]. Joule, 2023, 7(10): 2228-2244.
[20]. Ke X, Wang Y, Dai L, et al. Cell failures of all-solid-state lithium metal batteries with inorganic solid electrolytes: Lithium dendrites[J]. Energy Storage Materials, 2020, 33: 309-328.
[21]. Chen X R, Chen X, Yan C, et al. Role of lithiophilic metal sites in lithium metal anodes[J]. Energy & Fuels, 2021, 35(15): 12746-12752.
[22]. Ma Q, Zheng Y, Luo D, et al. 2D materials for all‐solid‐state lithium batteries[J]. Advanced Materials, 2022, 34(16): 2108079.
[23]. Huang S, Yang H, Hu J, et al. Early lithium plating behavior in confined nanospace of 3D lithiophilic carbon matrix for stable solid‐state lithium metal batteries[J]. Small, 2019, 15(43): 1904216.
[24]. Wen J, Huang Y, Duan J, et al. Highly adhesive Li-BN nanosheet composite anode with excellent interfacial compatibility for solid-state Li metal batteries[J]. ACS nano, 2019, 13(12): 14549-14556.
[25]. Feng S, Yeerella R H, Zhou J, et al. Homogenizing interfacial stress by nanoporous metal current collector to enable stable all-solid-state Li metal battery[J]. ACS Energy Letters, 2024, 9(2): 748-757.
[26]. Sun Z, Liu M, Zhu Y, et al. Issues concerning interfaces with inorganic solid electrolytes in all-solid-state lithium metal batteries[J]. Sustainability, 2022, 14(15): 9090.
[27]. Kong W, Wang S, Liu H, et al. Compositional Engineering of Lithium Metal Anode for High‐Performance Garnet Type Solid‐State Lithium Battery[J]. Small Methods, 2024: 2400910.
[28]. Wan H, Zhang B, Liu S, et al. Interface Design for High‐Performance All‐Solid‐State Lithium Batteries[J]. Advanced Energy Materials, 2024, 14(19): 2303046
[29]. Zeng Y, Ouyang B, Liu J, et al. High-entropy mechanism to boost ionic conductivity[J]. Science, 2022, 378(6626): 1320-1324.
[30]. Huang W Z, Liu Z Y, Xu P, et al. High-areal-capacity anode-free all-solid-state lithium batteries enabled by interconnected carbon-reinforced ionic-electronic composites[J]. Journal of Materials Chemistry A, 2023, 11(24): 12713-12718.
[31]. Liu Z, Huang W, Xiao Y, et al. Nanocomposite current collectors for anode-free all-solid-state lithium batteries[J]. Acta Physico-Chimica Sinica, 2024, 40(3): 2305040.
Cite this article
Bao,Z. (2025). Interfacial Engineering in Solid-State Lithium Metal Batteries: Degradation Mechanisms and Dynamic Regulation Strategies. Theoretical and Natural Science,109,8-17.
Data availability
The datasets used and/or analyzed during the current study will be available from the authors upon reasonable request.
Disclaimer/Publisher's Note
The statements, opinions and data contained in all publications are solely those of the individual author(s) and contributor(s) and not of EWA Publishing and/or the editor(s). EWA Publishing and/or the editor(s) disclaim responsibility for any injury to people or property resulting from any ideas, methods, instructions or products referred to in the content.
About volume
Volume title: Proceedings of CONF-MPCS 2025 Symposium: Leveraging EVs and Machine Learning for Sustainable Energy Demand Management
© 2024 by the author(s). Licensee EWA Publishing, Oxford, UK. This article is an open access article distributed under the terms and
conditions of the Creative Commons Attribution (CC BY) license. Authors who
publish this series agree to the following terms:
1. Authors retain copyright and grant the series right of first publication with the work simultaneously licensed under a Creative Commons
Attribution License that allows others to share the work with an acknowledgment of the work's authorship and initial publication in this
series.
2. Authors are able to enter into separate, additional contractual arrangements for the non-exclusive distribution of the series's published
version of the work (e.g., post it to an institutional repository or publish it in a book), with an acknowledgment of its initial
publication in this series.
3. Authors are permitted and encouraged to post their work online (e.g., in institutional repositories or on their website) prior to and
during the submission process, as it can lead to productive exchanges, as well as earlier and greater citation of published work (See
Open access policy for details).