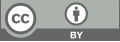
Accelerating Vaccine Development: Plug-and-Play Platforms for Emerging Infectious Diseases
- 1 The Stony Brook School, Stony Brook, NY 11790, USA
* Author to whom correspondence should be addressed.
Abstract
Emerging pathogens underscore an urgent need for rapidly developed vaccines to minimize mortality and societal disruption. Traditional vaccine development requires time spans of years, making it ill-suited to fast-evolving viruses that can overwhelm healthcare systems and economies. In response, plug-and-play vaccine platforms offer a more agile solution. By reusing proven backbones, they reduce the repetitive safety and production steps otherwise required for each new pathogen, thus accelerating both regulatory approval and large-scale manufacturing. In parallel, artificial intelligence and computational tools enable faster antigen and epitope identification, more accurate immune response modeling, and improved vaccine design. These innovations have already shortened timelines and enhanced efficacy.
Keywords
Vaccine, vaccine platforms, mRNA, viral vector machine, recombinant protein
[1]. Ukoaka, B. M., Okesanya, O. J., Daniel, F. M., Ahmed, M., Udam, N. G., Wagwula, P. M., Adigun, O. A., Udoh, R. A., Peter, I. G., & Lawal, H. (2024). Updated WHO list of emerging pathogens for a potential future pandemic: Implications for public health and global preparedness. Le Infezioni in Medicina: Rivista Periodica Di Eziologia, Epidemiologia, Diagnostica, Clinica e Terapia Delle Patologie Infettive, 4(32). https://doi.org/10.53854/liim-3204-5
[2]. Mangen, J. (2024). Vaccines in the Era of Emerging Infectious Diseases: Immune Mechanisms and Innovations. https://doi.org/10.59298/nijpp/2024/537110
[3]. Mylavarapu, S. G. (2024). COVID-19 Vaccine Impact and Future Threats From Other Pandemics. 2(5), 014–019. https://doi.org/10.69613/h561pp34
[4]. Chavda, V. P., Bezbaruah, R., Deka, K., Nongrang, L., & Kalita, T. (2022). The Delta and Omicron variants of SARS-CoV-2: what we know so far. Vaccines, 10(11), 1926.
[5]. Poria, R., Kala, D., Nagraik, R., Dhir, Y., Dhir, S., Singh, B., Kaushik, N. K., Noorani, Md. S., Kaushal, A., & Gupta, S. (2023). Vaccine development: Current trends and technologies. Life Science. https://doi.org/10.1016/j.lfs.2023.122331
[6]. Suzuki, M., & Yang, S. (2022). Political economy of vaccine diplomacy: explaining varying strategies of China, India, and Russia’s COVID-19 vaccine diplomacy. Review of International Political Economy, 30(3), 865–890. https://doi.org/10.1080/09692290.2022.2074514
[7]. Leunda, A., & Pauwels, K. (2019). GMO Regulatory Aspects of Novel Investigational Vaccine Candidates. IntechOpen. https://doi.org/10.5772/INTECHOPEN.85341
[8]. Ao, D., He, X., Liu, J., & Xu, L. (2023). Strategies for the development and approval of COVID-19 vaccines and therapeutics in the post-pandemic period. Signal Transduction and Targeted Therapy, 8. https://doi.org/10.1038/s41392-023-01724-w
[9]. Sil, D., Gautam, S., Saxena, S., Joshi, S., Kumar, D., Mehta, A., Jindal, P., Sharma, S., Pandey, P., & Singh, A. (2024). Comprehensive Analysis of Omicron Subvariants: EG.5 Rise, Vaccination Strategies, and Global Impact. Current Drug Targets. https://doi.org/10.2174/0113894501296586240430061915
[10]. Andey, T., Soni, S., & Modi, S. R. (2024). Conventional vaccination methods: Inactivated and live attenuated vaccines (pp. 37–50). Elsevier BV. https://doi.org/10.1016/b978-0-443-18564-9.00030-8
[11]. Xu, J., Yang, H., Zhang, X., Gao, M., Wang, L., & Sun, J. (2021). Recombinant viral vector, immunogenic composition comprising same and use thereof.
[12]. Hajek, A. E., & Goettel, M. S. (2000). Guidelines for Evaluating Effects of Entomopathogens on Non-target Organisms (pp. 847–868). Springer, Dordrecht. https://doi.org/10.1007/978-94-017-1547-8_38
[13]. Mikolajczyk, K., Bereznicka, A., Szymczak-Kulus, K., Haczkiewicz-Lesniak, K., Szulc, B., Olczak, M., Rossowska, J., Majorczyk, E., Kapczyńska, K., Bovin, N. V., Lisowska, M., Kaczmarek, R., Miazek, A., & Czerwinski, M. (2021). Missing the sweet spot: one of the two N-glycans on human Gb3/CD77 synthase is expendable. Glycobiology, 31(9), 1145–1162. https://doi.org/10.1093/GLYCOB/CWAB041
[14]. Li, L., Yang, C., Zhao, Z., Xu, B., Zheng, M., Zhang, C., Min, Z., Guo, J., & Rong, R. (2015). Skewed T-helper (Th)1/2- and Th17/T regulatory‑cell balances in patients with renal cell carcinoma. Molecular Medicine Reports, 11(2), 947–953. https://doi.org/10.3892/MMR.2014.2778
[15]. Avakian, A. P., Poston, R. M., Kong, F., Van Kampen, K. R., & Tang, D. C. (2007). Automated mass immunization of poultry: the prospect for nonreplicating human adenovirus-vectored in ovo vaccines. Expert Review of Vaccines, 6(3), 457–465. https://doi.org/10.1586/14760584.6.3.457
[16]. Vroegindewey, G. (2021). National Veterinary Services Roles and Responsibilities in Preparing for and Responding to Nuclear and Radiological Emergencies (pp. 1–11). Springer, Berlin, Heidelberg. https://doi.org/10.1007/978-3-662-63021-1_1
[17]. Li, X., Su, J., Guo, S., Yang, D., Sai, W., Qiu, X., Zhao, X., Wang, L., Wang, T., & Li, M. (2024). Platform Technology in Global Vaccine Regulation: Development, Applications, and Regulatory Strategies with Insights from China. Vaccines, 12(12), 1436. https://doi.org/10.3390/vaccines12121436
[18]. Jeon, J.-H., & Kim, E. Y. (2025). Exploring Future Pandemic Preparedness Through the Development of Preventive Vaccine Platforms and the Key Roles of International Organizations in a Global Health Crisis. Vaccines, 13(1), 56. https://doi.org/10.3390/vaccines13010056
[19]. Qin-sheng, Y. (2008). Pro-sequence Engineering. Pharmaceutical Biotechnology. https://en.cnki.com.cn/Article_en/CJFDTOTAL-YWSW200803017.htm
[20]. Mann, S. (2020). Creation of the Minority Genetic Professionals Network to increase diversity in the genetics work force. Journal of Genetic Counseling, 29(2), 202–205. https://doi.org/10.1002/JGC4.1248
[21]. Colak, E., Leslie, A., Zausmer, K., Khatamzas, E., Kubarenko, A. V., Pichulik, T., Klimosch, S. N., Mayer, A., Siggs, O. M., Hector, A., Fischer, R., Klesser, B., Rautanen, A., Frank, M., Hill, A. V. S., Manoury, B., Beutler, B., Hartl, D., Simmons, A., & Weber, A. N. R. (2014). RNA and Imidazoquinolines Are Sensed by Distinct TLR7/8 Ectodomain Sites Resulting in Functionally Disparate Signaling Events. Journal of Immunology, 192(12), 5963–5973. https://doi.org/10.4049/JIMMUNOL.1303058
[22]. Blois, M. (2023). Partners to scale cell-free biomanufacturing. C&EN Global Enterprise, 101(14), 9. https://doi.org/10.1021/cen-10114-buscon14
[23]. Shockney, L. D. (2015). Good Manufacturing Practice. https://jons-online.com/issues/2021/october-2021-vol-12-no-10?view=article&secid=65:conquering-the-cancer-care-continuum-series-three-first-issue&artid=1298:good-manufacturing-practice
[24]. Tan, T. J. C., Verma, A. K., Odle, A. E., Lei, R., Meyerholz, D. K., Matreyek, K. A., Perlman, S., Wong, L.-Y. R., & Wu, N. C. (2024). Evidence of antigenic drift in the fusion machinery core of SARS-CoV-2 spike. Proceedings of the National Academy of Sciences of the United States of America, 121(15), e2317222121. https://doi.org/10.1073/pnas.2317222121
[25]. Gupta, A., Satapathy, T., Pradhan, B., Sen, K., Sahu, S., Sahu, A. K., Bhardwaj, S. K., & Khan, M. A. (2024). Experimental Animal Models for Influenza/Flu Virus Vaccine Development. Journal of Drug Delivery and Therapeutics, 14(2), 192–204. https://doi.org/10.22270/jddt.v14i2.6362
[26]. Razim, A., Górska, S., & Myc, A. (2022). Effective mucosal vaccines - opportunities and challenges. Postepy Biochemii, 68(2), 179–188. https://doi.org/10.18388/pb.2021_439
[27]. Bahakel, H., Spieker, A. J., Hayek, H., Schuster, J. E., Hamdan, L., Dulek, D. E., Kitko, C. L., Stopczynski, T., Batarseh, E., Haddadin, Z., Stewart, L. S., Stahl, A., Potter, M., Rahman, H., Amarin, J. Z., Kalams, S. A., Bocchini, C., Moulton, E. A., Coffin, S., … Smith, H. (2024). Immunogenicity and Reactogenicity of High-dose or Standard-dose Influenza Vaccine in a Second Consecutive Influenza Season. The Journal of Infectious Diseases. https://doi.org/10.1093/infdis/jiae454
[28]. Germano, M. J., Mackern-Oberti, J. P., Vitório, J. G., Duarte, M. C., Pimenta, D. C., Sánchez, M. V., Bruna, F., Lozano, E., Fernandes, A., & Cargnelutti, D. E. (2022). Identification of Immunodominant Antigens From a First-Generation Vaccine Against Cutaneous Leishmaniasis. Frontiers in Immunology, 13. https://doi.org/10.3389/fimmu.2022.825007
[29]. Forster Iii, J., Nandi, D., & Kulkarni, A. (2022). mRNA-carrying lipid nanoparticles that induce lysosomal rupture activate NLRP3 inflammasome and reduce mRNA transfection efficiency. Biomaterials Science, 10(19), 5566–5582. https://doi.org/10.1039/d2bm00883a
[30]. Kuehn, M., LaMori, J., DeMartino, J. K., Mesa-Frias, M., Doran, J., Korrapati, L., Bhojwani, R., Lefebvre, P., & Kirson, N. Y. (2022). Assessing barriers to access and equity for COVID-19 vaccination in the US. BMC Public Health, 22(1). https://doi.org/10.1186/s12889-022-14636-1
[31]. Euzen, A. (2022). The Silver Bullet: A Safe and Efficient Attenuated Vaccine for Viral Diseases Based on Biothermodynamics. https://doi.org/10.20944/preprints202212.0454.v1
[32]. Ueno, S., & Nemoto, N. (2012). cDNA display: rapid stabilization of mRNA display (Vol. 805, pp. 113–135). Springer, New York, NY. https://doi.org/10.1007/978-1-61779-379-0_8
[33]. Alsaggaf, I., & Wan, C. (2024). Functional yeast promoter sequence design using temporal convolutional generative language models. https://doi.org/10.1101/2024.10.22.619701
[34]. Glorioso, J. C., Cohen, J. B., Miyagawa, Y., Krisky, D., Wechuck, J. B., & Wolfe, D. (2014). Non-toxic hsv vectors for efficient gene delivery applications and complementing cells for their production. https://patents.google.com/patent/WO2015009952A1/en
[35]. Chang, S. Y., Bisht, A., Faysman, K., Schiller, G. J., Uslan, D. Z., Uslan, D. Z., & Multani, A. (2021). Vaccine-Associated Measles in a Hematopoietic Cell Transplant Recipient: Case Report and Comprehensive Review of the Literature. Open Forum Infectious Diseases, 8(8). https://doi.org/10.1093/OFID/OFAB326
[36]. Naseri, R., Navabi, S. J., Samimi, Z., Mishra, A. P., Nigam, M., Chandra, H., Olatunde, A., Tijjani, H., Morais-Urano, R. P., & Farzaei, M. H. (2020). Targeting Glycoproteins as a therapeutic strategy for diabetes mellitus and its complications. Tehran University of Medical Sciences, 28(1), 333–358. https://doi.org/10.1007/S40199-020-00327-Y
[37]. Żak, A., Żebrowska-Różańska, P., Bajzert, J., Siwińska, N., Madej, J. P., Kaleta-Kuratewicz, K., Bochen, P., Łaczmański, Ł., & Chełmońska-Soyta, A. (2024). Comparison and characterization of the bacterial microbiota and SIgA production in different gastrointestinal segments in horses. Veterinary Research Communications. https://doi.org/10.1007/s11259-024-10489-8
[38]. Gupta, S., Padhi, B. K., Gandhi, A. P., Satapathy, P., Kukreti, N., Rustagi, S., Khatib, M. N., Gaidhane, A., & Zahiruddin, Q. S. (2024). Efficacy of Single-Dose HPV Vaccine Beyond Multiple Doses: A Systematic Review and Meta-Analysis of Available Evidence. https://doi.org/10.2139/ssrn.4716777
[39]. Baldo, A., van den Akker, E., Bergmans, H. E., Lim, F., & Pauwels, K. (2014). General considerations on the biosafety of virus-derived vectors used in gene therapy and vaccination. Current Gene Therapy, 13(6), 385–394. https://doi.org/10.2174/15665232113136660005
[40]. Filaire, F., Bertran, K., Gaide, N., Valle, R., Secula, A., Perlas, A., Foret‐Lucas, C., Nofrarías, M., Cantero, G., Croville, G., Majó, N., & Guérin, J. (2024). Viral shedding and environmental dispersion of two clade 2.3.4.4b H5 high pathogenicity avian influenza viruses in experimentally infected mule ducks: implications for environmental sampling. Veterinary Research, 55(1). https://doi.org/10.1186/s13567-024-01357-z
[41]. Joseph, J., Mathew, J., & Alexander, J. J. (2024). Scaffold Proteins in Autoimmune Disorders. Current Rheumatology Reviews, 20(1), 14–26. https://doi.org/10.2174/1573397119666230904151024
[42]. Su, W. W., & Han, Z. (2013). Self-Assembled Synthetic Protein Scaffolds: Biosynthesis and Applications. 50(28), 23–29. https://doi.org/10.1149/05028.0023ECST
[43]. Liu, W., Halverson, J. D., Tian, Y., Tkachenko, A. V., & Gang, O. (2016). Self-organized architectures from assorted DNA-framed nanoparticles. Nature Chemistry, 8(9), 867–873. https://doi.org/10.1038/NCHEM.2540
[44]. Principles of Biophysical and Biochemical Characterization of Root Vegetables’ Bioactive Proteins. (2023). IntechOpen eBooks. https://doi.org/10.5772/intechopen.107986
[45]. Wamhoff, E.-C., Ronsard, L., Feldman, J., Knappe, G. A., Hauser, B. M., Romanov, A. M., Lam, E. C., St. Denis, K., Boucau, J., Barczak, A. K., Balazs, A. B., Schmidt, A. G., Lingwood, D., & Bathe, M. (2022). Enhancing antibody responses by multivalent antigen display on thymus-independent DNA origami scaffolds. bioRxiv. https://doi.org/10.1101/2022.08.16.504128
[46]. Zhou, P., Luo, Q., Lin, Y., Chen, L., Li, S., Zhou, G., Ji, X., & He, Z. (2012). Immunoassays with protein misfolding cycle amplification: a platform for ultrasensitive detection of antigen. Analytical Chemistry, 84(17), 7343–7349. https://doi.org/10.1021/AC300805U
[47]. Wang, J., Xiangdong, H., Jialin, Q., Rong, K., Yingbo, L., Chen, W., & Mingsheng, L. (2019). Column-based storage method and system for timing data and query method and system for timing data.
[48]. Nguyen, B., & Tolia, N. H. (2021). Protein-based antigen presentation platforms for nanoparticle vaccines. 6(1), 70. https://doi.org/10.1038/S41541-021-00330-7
[49]. Fernández-Quintero, M. L., Kroell, K. B., Heiss, M. C., Loeffler, J. R., Quoika, P. K., Waibl, F., Bujotzek, A., Moessner, E., Georges, G., & Liedl, K. R. (2020). Surprisingly Fast Interface and Elbow Angle Dynamics of Antigen-Binding Fragments. Frontiers in Molecular Biosciences, 7, 609088. https://doi.org/10.3389/FMOLB.2020.609088
[50]. Leontieva, G., Kramskaya, T., Grabovskaya, K. B., Gupalova, T., Dmitriev, A., & Suvorov, A. (2023). Recombinant vaccine candidates with integrated adjuvants provide stimulation of an effective immune response against bacterial infections. Russian Journal for Personalized Medicine, 2(6), 64–77. https://doi.org/10.18705/2782-3806-2022-2-6-64-77
[51]. Kim, Y.-I., Kim, D., Yu, K.-M., Seo, H. D., Lee, S.-A., Casel, M. A. B., Jang, S.-G., Kim, S., Jung, W., Lai, C.-J., Choi, Y. K., & Jung, J. U. (2021). Development of spike receptor-binding domain nanoparticle as a vaccine candidate against SARS-CoV-2 infection in ferrets. bioRxiv. https://doi.org/10.1101/2021.01.28.428743
[52]. Martinez, G. S., Dutt, M., Kelvin, D. J., & Kumar, A. (2024). PoxiPred: An Artificial-Intelligence-Based Method for the Prediction of Potential Antigens and Epitopes to Accelerate Vaccine Development Efforts against Poxviruses. Biology, 13(2), 125. https://doi.org/10.3390/biology13020125
[53]. Shi, X., Tao, Y., & Lin, S.-C. (2024). Deep Neural Network-Based Prediction of B-Cell Epitopes for SARS-CoV and SARS-CoV-2: Enhancing Vaccine Design through Machine Learning. https://doi.org/10.48550/arxiv.2412.00109
[54]. Vaccine Development Through Reverse Vaccinology Using Artificial Intelligence and Machine Learning Approach (pp. 33–49). (2022). Elsevier eBooks. https://doi.org/10.1016/b978-0-323-85844-1.00006-4
[55]. Vaccine Development Through Reverse Vaccinology Using Artificial Intelligence and Machine Learning Approach (pp. 33–49). (2022). Elsevier eBooks. https://doi.org/10.1016/b978-0-323-85844-1.00006-4
[56]. Panchariya, D. C., Karthic, A., Singh, S. P., Mani, A., Chawade, A., & Kushwaha, S. (2024). Vaccine design and development: Exploring the interface with computational biology and AI. International Reviews of Immunology, 1–20. https://doi.org/10.1080/08830185.2024.2374546
[57]. Imani, S., Li, X., Chen, K., Maghsoudloo, M., Kaboli, P. J., Hashemi, M., Khoushab, S., & Li, X. (2025). Computational biology and artificial intelligence in mRNA vaccine design for cancer immunotherapy. Frontiers in Cellular and Infection Microbiology, 14. https://doi.org/10.3389/fcimb.2024.1501010
[58]. Liu, D.-X., Xu, Y., & Qian, C. (2024). Peptide Vaccine Design by Evolutionary Multi-Objective Optimization. https://doi.org/10.24963/ijcai.2024/770
[59]. Klein, J., Sprague, D., Lane, M., Hart, M. G., Petrillo, O., Faria do Valle, I., Ferguson, A., Allen, A., Jooss, K., & Dhanik, A. (2024). Abstract 904: AI platform provides an EDGE and enables state-of-the-art identification of peptide-HLAs for the development of T cell inducing vaccines. Cancer Research. https://doi.org/10.1158/1538-7445.am2024-904
[60]. Tomic, I. (2024). Immunaut: Immunophenotype Analysis with Machine Learning for LAIV Vaccine Response Prediction. Journal of Immunology, 212(1_Supplement), 0879_6158. https://doi.org/10.4049/jimmunol.212.supp.0879.6158
Cite this article
Yang,K. (2025). Accelerating Vaccine Development: Plug-and-Play Platforms for Emerging Infectious Diseases. Theoretical and Natural Science,111,1-9.
Data availability
The datasets used and/or analyzed during the current study will be available from the authors upon reasonable request.
Disclaimer/Publisher's Note
The statements, opinions and data contained in all publications are solely those of the individual author(s) and contributor(s) and not of EWA Publishing and/or the editor(s). EWA Publishing and/or the editor(s) disclaim responsibility for any injury to people or property resulting from any ideas, methods, instructions or products referred to in the content.
About volume
Volume title: Proceedings of ICBioMed 2025 Symposium: AI for Healthcare: Advanced Medical Data Analytics and Smart Rehabilitation
© 2024 by the author(s). Licensee EWA Publishing, Oxford, UK. This article is an open access article distributed under the terms and
conditions of the Creative Commons Attribution (CC BY) license. Authors who
publish this series agree to the following terms:
1. Authors retain copyright and grant the series right of first publication with the work simultaneously licensed under a Creative Commons
Attribution License that allows others to share the work with an acknowledgment of the work's authorship and initial publication in this
series.
2. Authors are able to enter into separate, additional contractual arrangements for the non-exclusive distribution of the series's published
version of the work (e.g., post it to an institutional repository or publish it in a book), with an acknowledgment of its initial
publication in this series.
3. Authors are permitted and encouraged to post their work online (e.g., in institutional repositories or on their website) prior to and
during the submission process, as it can lead to productive exchanges, as well as earlier and greater citation of published work (See
Open access policy for details).