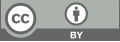
Regulation of Microglial Polarization through the NF-kB Pathway by activating Rho/ROCK Pathway induced by LPS Priming in the 5xFAD Mouse Model of AD
- 1 Graduate school, Hebei Medical University, Shijiazhuang, China
- 2 College of Biological Sciences, University of California, Davis; Davis, United States
* Author to whom correspondence should be addressed.
Abstract
The symptoms of Alzheimer's disease (AD) are the deposition of beta-amyloid plaques and chronic neuroinflammation caused by microglia. Its pathological process is closely related to microglia. Microglia have innated immune memory (IIM) and have different roles that vary with animal models of neurodegenerative diseases such as AD. However, how IIM-mediated microglia function is well validated in the 5*FAD model. We want to know if lipopolysaccharide (LPS)-induced IIM in the preclinical period of AD alters microglia polarization. We did this by injecting LPS into 5*FAD mice at 6 weeks (before plaque formation). After 140 days, we assessed microglia polarization and activation of the Rho/ROCK pathway and NF-κB pathway in 5*FAD-initiated and non-initiated mice. Furthermore, the activation of them in microglia was also evaluated. ROCK2 was overexpressed in primary microglia by lentivirus transfection. Then, ROCK2 overexpression model was constructed by CRISPR/Cas9 based on 5*FAD mice. Cross with Tmem119-CreERT2 mice to obtain microglia overexpressing ROCK2 mice. The activation of microglia, and these two pathways were evaluated after LPS-induced IIM. Our expected result is that LPS-induced IIM changes microglial polarization from M1 to M2 by the inhibition of them. The paper only provides theoretical experiment design and possible results about alterations in microglia polarization and their mechanisms after LPS priming stimulates innate immune memory, which needs further research in its mechanisms. Suggestions for more long-term effects and potential therapeutic applications in humans also has been listed for reference
Keywords
Alzheimer’s disease, LPS, Priming, innate immune memory, microglial, polarization, Rho/ROCk pathway, NF-κB pathway
[1]. 2022 Alzheimer's disease facts and figures. Alzheimers Dement, 2022. 18(4): p. 700-789.
[2]. Hardy, J., A hundred years of Alzheimer's disease research. Neuron, 2006. 52(1): p. 3-13.
[3]. Kinney, J.W., et al., Inflammation as a central mechanism in Alzheimer's disease. Alzheimers Dement (N Y), 2018. 4: p. 575-590.
[4]. Heppner, F.L., R.M. Ransohoff, and B. Becher, Immune attack: the role of inflammation in Alzheimer disease. Nat Rev Neurosci, 2015. 16(6): p. 358-72.
[5]. Zhang, W., et al., Role of neuroinflammation in neurodegeneration development. Signal Transduct Target Ther, 2023. 8(1): p. 267.
[6]. Cai, Y., et al., Microglia in the Neuroinflammatory Pathogenesis of Alzheimer's Disease and Related Therapeutic Targets. Front Immunol, 2022. 13: p. 856376.
[7]. Ousman, S.S. and P. Kubes, Immune surveillance in the central nervous system. Nat Neurosci, 2012. 15(8): p. 1096-101.
[8]. Salter, M.W. and B. Stevens, Microglia emerge as central players in brain disease. Nat Med, 2017. 23(9): p. 1018-1027.
[9]. Liu, L.R., et al., Interaction of Microglia and Astrocytes in the Neurovascular Unit. Front Immunol, 2020. 11: p. 1024.
[10]. Askew, K., et al., Coupled Proliferation and Apoptosis Maintain the Rapid Turnover of Microglia in the Adult Brain. Cell Rep, 2017. 18(2): p. 391-405.
[11]. Fuger, P., et al., Microglia turnover with aging and in an Alzheimer's model via long-term in vivo single-cell imaging. Nat Neurosci, 2017. 20(10): p. 1371-1376.
[12]. Reu, P., et al., The Lifespan and Turnover of Microglia in the Human Brain. Cell Rep, 2017. 20(4): p. 779-784.
[13]. Candore, G., et al., Major histocompatibility complex and sporadic Alzheimer's disease: a critical reappraisal. Exp Gerontol, 2004. 39(4): p. 645-52.
[14]. Crous-Bou, M., et al., Alzheimer's disease prevention: from risk factors to early intervention. Alzheimers Res Ther, 2017. 9(1): p. 71.
[15]. Silva, M.V.F., et al., Alzheimer's disease: risk factors and potentially protective measures. J Biomed Sci, 2019. 26(1): p. 33.
[16]. Lenz, K.M. and L.H. Nelson, Microglia and Beyond: Innate Immune Cells As Regulators of Brain Development and Behavioral Function. Front Immunol, 2018. 9: p. 698.
[17]. Zhao, Y., L. Cong, and W.J. Lukiw, Lipopolysaccharide (LPS) Accumulates in Neocortical Neurons of Alzheimer's Disease (AD) Brain and Impairs Transcription in Human Neuronal-Glial Primary Co-cultures. Front Aging Neurosci, 2017. 9: p. 407.
[18]. Wang, X. and P.J. Quinn, Lipopolysaccharide: Biosynthetic pathway and structure modification. Prog Lipid Res, 2010. 49(2): p. 97-107.
[19]. Hu, N. and Y. Zhang, TLR4 knockout attenuated high fat diet-induced cardiac dysfunction via NF-kappaB/JNK-dependent activation of autophagy. Biochim Biophys Acta Mol Basis Dis, 2017. 1863(8): p. 2001-2011.
[20]. Hamilton, C., et al., NLRP3 Inflammasome Priming and Activation Are Regulated by a Phosphatidylinositol-Dependent Mechanism. Immunohorizons, 2022. 6(8): p. 642-659.
[21]. Chen, S., et al., Macrophages in immunoregulation and therapeutics. Signal Transduct Target Ther, 2023. 8(1): p. 207.
[22]. Cunningham, C. and E. Hennessy, Co-morbidity and systemic inflammation as drivers of cognitive decline: new experimental models adopting a broader paradigm in dementia research. Alzheimers Res Ther, 2015. 7(1): p. 33.
[23]. Neher, J.J. and C. Cunningham, Priming Microglia for Innate Immune Memory in the Brain. Trends Immunol, 2019. 40(4): p. 358-374.
[24]. Wendeln, A.C., et al., Innate immune memory in the brain shapes neurological disease hallmarks. Nature, 2018. 556(7701): p. 332-338.
[25]. Yang, Y., et al., LPS priming before plaque deposition impedes microglial activation and restrains Abeta pathology in the 5xFAD mouse model of Alzheimer's disease. Brain Behav Immun, 2023. 113: p. 228-247.
[26]. Song, G.J. and K. Suk, Pharmacological Modulation of Functional Phenotypes of Microglia in Neurodegenerative Diseases. Front Aging Neurosci, 2017. 9: p. 139.
[27]. Xu, Y., et al., Melatonin attenuates choroidal neovascularization by regulating macrophage/microglia polarization via inhibition of RhoA/ROCK signaling pathway. J Pineal Res, 2020. 69(1): p. e12660.
[28]. Koch, J.C., et al., ROCK inhibition in models of neurodegeneration and its potential for clinical translation. Pharmacol Ther, 2018. 189: p. 1-21.
[29]. Zandi, S., et al., ROCK-isoform-specific polarization of macrophages associated with age-related macular degeneration. Cell Rep, 2015. 10(7): p. 1173-86.
[30]. Boza-Serrano, A., et al., Galectin-3, a novel endogenous TREM2 ligand, detrimentally regulates inflammatory response in Alzheimer's disease. Acta Neuropathol, 2019. 138(2): p. 251-273.
[31]. Imai, Y., et al., A novel gene iba1 in the major histocompatibility complex class III region encoding an EF hand protein expressed in a monocytic lineage. Biochem Biophys Res Commun, 1996. 224(3): p. 855-62.
[32]. Heneka, M.T., M.P. Kummer, and E. Latz, Innate immune activation in neurodegenerative disease. Nat Rev Immunol, 2014. 14(7): p. 463-77.
[33]. Hendrickx, D.A., et al., Selective upregulation of scavenger receptors in and around demyelinating areas in multiple sclerosis. J Neuropathol Exp Neurol, 2013. 72(2): p. 106-18.
[34]. Tedesco, S., et al., Phenotypic activation and pharmacological outcomes of spontaneously differentiated human monocyte-derived macrophages. Immunobiology, 2015. 220(5): p. 545-54.
[35]. Shabo, I. and J. Svanvik, Expression of macrophage antigens by tumor cells. Adv Exp Med Biol, 2011. 714: p. 141-50.
[36]. Sica, A. and A. Mantovani, Macrophage plasticity and polarization: in vivo veritas. J Clin Invest, 2012. 122(3): p. 787-95.
[37]. Li, P., et al., Melatonin regulates microglial polarization to M2 cell via RhoA/ROCK signaling pathway in epilepsy. Immun Inflamm Dis, 2023. 11(6): p. e900.
[38]. Szasz, T. and R.C. Webb, Rho-Mancing to Sensitize Calcium Signaling for Contraction in the Vasculature: Role of Rho Kinase. Adv Pharmacol, 2017. 78: p. 303-322.
[39]. Lu, W., J. Wen, and Z. Chen, Distinct Roles of ROCK1 and ROCK2 on the Cerebral Ischemia Injury and Subsequently Neurodegenerative Changes. Pharmacology, 2020. 105(1-2): p. 3-8.
[40]. Chen, J., et al., Inhibition of AGEs/RAGE/Rho/ROCK pathway suppresses non-specific neuroinflammation by regulating BV2 microglial M1/M2 polarization through the NF-kappaB pathway. J Neuroimmunol, 2017. 305: p. 108-114.
[41]. Saijo, K. and C.K. Glass, Microglial cell origin and phenotypes in health and disease. Nat Rev Immunol, 2011. 11(11): p. 775-87.
[42]. Zheng, J., et al., Ceria nanoparticles ameliorate white matter injury after intracerebral hemorrhage: microglia-astrocyte involvement in remyelination. J Neuroinflammation, 2021. 18(1): p. 43.
[43]. Hoffmann, A. and D. Baltimore, Circuitry of nuclear factor kappaB signaling. Immunol Rev, 2006. 210: p. 171-86.
[44]. Qian, X., et al., Vitamin D attenuates myocardial ischemia-reperfusion injury by inhibiting inflammation via suppressing the RhoA/ROCK/NF-kB pathway. Biotechnol Appl Biochem, 2019. 66(5): p. 850-857.
[45]. Hu, F., et al., Moderate extracellular acidification inhibits capsaicin-induced cell death through regulating calcium mobilization, NF-kappaB translocation and ROS production in synoviocytes. Biochem Biophys Res Commun, 2012. 424(1): p. 196-200.
[46]. Towriss, M., B. MacVicar, and A.V. Ciernia, Modelling Microglial Innate Immune Memory In Vitro: Understanding the Role of Aerobic Glycolysis in Innate Immune Memory. Int J Mol Sci, 2023. 24(10).
[47]. An, Y., et al., PL201, a Reported Rhamnoside Against Alzheimer's Disease Pathology, Alleviates Neuroinflammation and Stimulates Nrf2 Signaling. Front Immunol, 2020. 11: p. 162.
[48]. Rashid, K., K. Dannhausen, and T. Langmann, Testing for Known Retinal Degeneration Mutants in Mouse Strains. Methods Mol Biol, 2019. 1834: p. 45-58.
[49]. Eskandari-Sedighi, G., et al., Alzheimer's disease associated isoforms of human CD33 distinctively modulate microglial cell responses in 5XFAD mice. Mol Neurodegener, 2024. 19(1): p. 42.
[50]. Gustavsson, T., et al., Long-term effects of immunotherapy with a brain penetrating Abeta antibody in a mouse model of Alzheimer's disease. Alzheimers Res Ther, 2023. 15(1): p. 90.
[51]. Zhang, J.X., et al., Rescue of cognitive deficits in APP/PS1 mice by accelerating the aggregation of beta-amyloid peptide. Alzheimers Res Ther, 2019. 11(1): p. 106.
[52]. Chen, X.Z., Ye & Sadadcharam, Gaitri & Cui, Weili & Wang, J.H., Isolation, Purification, and Culture of Primary Murine Microglia Cells. Bioprotocol, 2013. 3.
[53]. Lu, W., Y. Wang, and J. Wen, The Roles of RhoA/ROCK/NF-kappaB Pathway in Microglia Polarization Following Ischemic Stroke. J Neuroimmune Pharmacol, 2024. 19(1): p. 19.
[54]. Ye, X., et al., Lipopolysaccharide induces neuroinflammation in microglia by activating the MTOR pathway and downregulating Vps34 to inhibit autophagosome formation. J Neuroinflammation, 2020. 17(1): p. 18.
[55]. Yan, Y., et al., Therapeutic potentials of the Rho kinase inhibitor Fasudil in experimental autoimmune encephalomyelitis and the related mechanisms. Metab Brain Dis, 2019. 34(2): p. 377-384.
[56]. Oblak, A.L., et al., Comprehensive Evaluation of the 5XFAD Mouse Model for Preclinical Testing Applications: A MODEL-AD Study. Front Aging Neurosci, 2021. 13: p. 713726.
Cite this article
Xu,Y.;You,K. (2025). Regulation of Microglial Polarization through the NF-kB Pathway by activating Rho/ROCK Pathway induced by LPS Priming in the 5xFAD Mouse Model of AD. Theoretical and Natural Science,73,228-241.
Data availability
The datasets used and/or analyzed during the current study will be available from the authors upon reasonable request.
Disclaimer/Publisher's Note
The statements, opinions and data contained in all publications are solely those of the individual author(s) and contributor(s) and not of EWA Publishing and/or the editor(s). EWA Publishing and/or the editor(s) disclaim responsibility for any injury to people or property resulting from any ideas, methods, instructions or products referred to in the content.
About volume
Volume title: Proceedings of the 4th International Conference on Biological Engineering and Medical Science
© 2024 by the author(s). Licensee EWA Publishing, Oxford, UK. This article is an open access article distributed under the terms and
conditions of the Creative Commons Attribution (CC BY) license. Authors who
publish this series agree to the following terms:
1. Authors retain copyright and grant the series right of first publication with the work simultaneously licensed under a Creative Commons
Attribution License that allows others to share the work with an acknowledgment of the work's authorship and initial publication in this
series.
2. Authors are able to enter into separate, additional contractual arrangements for the non-exclusive distribution of the series's published
version of the work (e.g., post it to an institutional repository or publish it in a book), with an acknowledgment of its initial
publication in this series.
3. Authors are permitted and encouraged to post their work online (e.g., in institutional repositories or on their website) prior to and
during the submission process, as it can lead to productive exchanges, as well as earlier and greater citation of published work (See
Open access policy for details).